
해양산업시설 배출 위험·유해물질 해양환경 영향평가; 안정동위원소 기반 생물 축적성 평가







초록
위험·유해물질(HNS, Harzardous and Noxious Substances)의 배출허용 기준치 설정과 관련하여, 해양산업시설 주변 방출되는 HNS의 현장기반 생물축적 정보는 수생태계 독성평가 데이터베이스(DB)를 구축하는데 중요하다. 본 연구에서는 안정동위원소 기법을 이용하여 해양생태계 내 먹이단계 구조를 우선적으로 결정하여, 생물영양단계 축적평가와 관련된 활용 가능성을 파악하고자 하였다. 전국 해양산업시설 주변(평택, 보령, 영광, 여수, 울산)에서 측정된 입자성 유기물질(POM) 내 질소 안정동위원소값(δ15N)은 지역 간 유의한 차이(울산-평택; 10.9‰, p<0.01)를 보였으며, 이는 각 지역 해양생태계 내 유입되는 유기물 공급 특성 차이가 반영된 것으로 판단된다. 이러한 배경 δ15N값의 변동성은 상위영양생물의 총 조직(bulk tissue)을 활용한 먹이단계(TP) 산출시 동일 해양생물 종임에도 서식지별 TP가 달리 산출될 수 있음을 의미한다. 이를 보완하기 위해 테스트베드(울산 온산만) 내 채집된 시료(POM, 동물플랑크톤, 어류) 내 특정 아미노산(페닐알라닌과 글루타민산)의 δ15N값을 추가 분석하였다. 이를 통해 동물플랑크톤-어류 내 전형적인 영양단계 분별계수(TDF; ~8‰)을 반영한 먹이사슬 구조 특징을 확인하였다. 하지만, 글로벌 TP 데이터베이스와의 비교시, 테스트베드 내 ΔTP 변동성(TPbulk-fishbase, TPAA—fishbase)은 통계적 유의한 차이(p>0.01)는 보이지 않았다. 따라서 테스트베드 내 두 방법을 사용한 TP 평가는 현장기반 HNS 생물축적성 규명을 위해 모두 적용 가능할 것으로 예상된다. 추후, 전국단위 수준에서 보다 신뢰성 있는 HNS 생물축적 자료를 도출하기 위해 해양산업시설 주변 다양한 해양생물 내 TP 자료 확보가 필요할 것으로 판단된다.
Abstract
With respect to the establishment of the effluent quality standard of harzardous and noxious substances (HNS), the information of in situ HNS bioaccumulation may be important for developing the database related to aquatic toxicity. In this study, our objectives is to determine the trophic position structure in marine ecosystem by using stable isotope techniques, and to estimate the potential applicability related to the trophic magnification of HNS. The nitrogen isotopic values (δ15N) of particulate organic matter (POM) at marine industrial facilities showed regionally significant difference (Ulsan-Pyeontaek; 10.9‰, p<0.01), indicating different contributions of POM sources transported into marine environments. Furthermore, variations of baseline δ15N values mean that the trophic position (TP) calculated between same marine species may depend on different regional conditions during TP estimation via bulk tissue. To complement these limitations, the δ15N analysis of specific amino acids (phenylalanine, glutamic acid) additionally applied for environmental materials (POM, zooplankton, fish) collected at test-bed (Onsan Bay, Ulsan). In this regard, we confirmed that food-chain structures formed within the test-bed might be closely related to typical trophic discrimination factor (TDF; ~8‰) occurred from zooplankton to fish. However, ΔTP variations at the test-bed showed no significant difference (p>0.01), compared to the global TP database. Thus, our results propose that the preliminary TP calculations using both methods may commonly apply for estimating in situ HNS bioaccumulation in the test-bed. As further studies, TP information among various marine organisms inhabiting nationwide marine industrial facilities may be necessary for more precisely estimations of HNS bioaccumulation.
Keywords:
Hazardous and Noxious Substance, Marine discharging assessment, Bioaccumulation, Stable isotope composition키워드:
위험유해물질, 배출영향평가, 생물축적, 안정동위원소1. 서 론
위험·유해물질로 불리는 HNS(harzardous and noxious substances)는 국제적으로 해양생태계에 해를 주는 물질(harmful substance; marine pollution classification (MARPOL) 73/78), 선박과 선원의 안전과 관련된 물질(dangerous goods; SOLAS 74), 해상운송되는 어떠한 물질, 원료, 품목에서 위험하거나 해로운 물질(HNS Convention)이라 정의하고 있다. 또한 oil pollution preparedness, response and cooperation(OPRC)-HNS 의정서는 HNS를 인간의 건강과 해양 생물에 해로운 영향을 미치며, 쾌적성을 손상시키거나 다른 합법적인 바다의 이용에 방해가 되는 물질로 정의하고 있다(Harold et al.[2014]; IMO[2000]). 우리나라의 경우, 2008년에 OPRC-HNS 의정서를 수용하여 HNS 해양사고에 대한 선제적 국가대응체제(선박안전법, 해양환경관리법, 위험물안전관리법, 유해화학물질관리법)를 구축 중에 있다.
국내 해상운송량의 약 19%를 차지하는 HNS(약 6천여종, 2억5천1백만톤)는 최근 10년간 약 66%의 증가추세인 것으로 파악되고 있다(MPSS[2015]). 더욱이, 국내 해양환경, 인명 및 재산피해를 줄 수 있는 HNS 유출사고가 지속적으로 증가되는 것을 감안할 때(Cho et al.[2013]; Lee and Jung[2013]; Lee and Oh[2014]), HNS의 종류와 거동특성을 효과적으로 관리할 수 있는 대응방안 마련이 시급히 필요한 실정이다. 이와 관련하여, 국내외 화학물질 관리 우선순위 선정기법을 토대로 위해성 기반의 HNS 관리 우선순위선정기법을 개발하여 데이터베이스화를 진행시킨바 있다(Kim et al.[2015]). 그러나 HNS 관리 우선순위선정기법 적용시 일부 만성독성 자료 등의 부재로 인해 기본값 및 추정치가 적용되었으며, 수생태 독성의 경우 해양 생물종에 대한 자료가 국내외적으로 빈약하여 환경 위해성 등의 정보를 구체적으로 반영하지 못하고 있다는 한계가 있다. 따라서, 다양한 HNS의 물리화학적 특성을 토대로 현장기반 유해성(독성, 생물축적)자료를 도출하여 보다 신뢰성 있는 자료 확보가 요구되는 실정이다. 특히, 생물 축적성 평가와 관련하여 질소 및 아미노산 안정동위원소비를 활용한 생물 영양단계 평가는 필수적이며, 이를 활용하여 유해물질의 생물축적계수를 산정한 바 있다(Won et al.[2018]; Kim et al.[2022]). 이에 본 연구에서는 합리적인 HNS 배출기준치 설정을 위해 안정동위원소 기법을 이용하여 해양생태계 먹이망을 체계적으로 평가하고자 하였다. 이를 통해 추후 해양산업시설 주변 현장기반 HNS 생물 축적 자료를 제공하고자 하였다.
2. 재료 및 방법
2.1 시료채집
해양생태계 내 먹이망 구조 파악 연구를 위해 우선적으로 전국에 위치한 해양산업시설 주변(평택, 보령, 영광, 여수, 울산)에서 표층 해수 채취 후(2021년 9월, Table 1 and Fig. 1), 실험실에서 미리 태운 25 mm 유리섬유 여과지(GF/F, pore size: 0.7 μm)로 여과하였다. 또한, 테스트베드(울산 온산만) 내에서 입자성 부유물질뿐만 아니라 동물플랑크톤(NORPAC네트, 망구 45 cm) 채집을 위해 바닥-표층(1 m 수심)에서 수직채집을 수행하였고, 채집된 시료는 드라이아이스를 이용하여 -80℃에서 냉동보관하여 실험실로 운반하였다(2021년 10월). 실험실에서 동물플랑크톤 시료는 냉장실에서 해동 후 해부현미경(V20, Zeiss, Germany) 상에서 동정 후 종별 100개체를 선별하여 유리병으로 옮겼다. 해양산업시설 주변 서식하는 주요 생물종 채집을 위해 통발을 이용하였으며, 3일 후 수거하여 채집된 생물종은 분석 전까지 냉동보관(< -20℃) 하였다(Table 2 and Fig. 1).
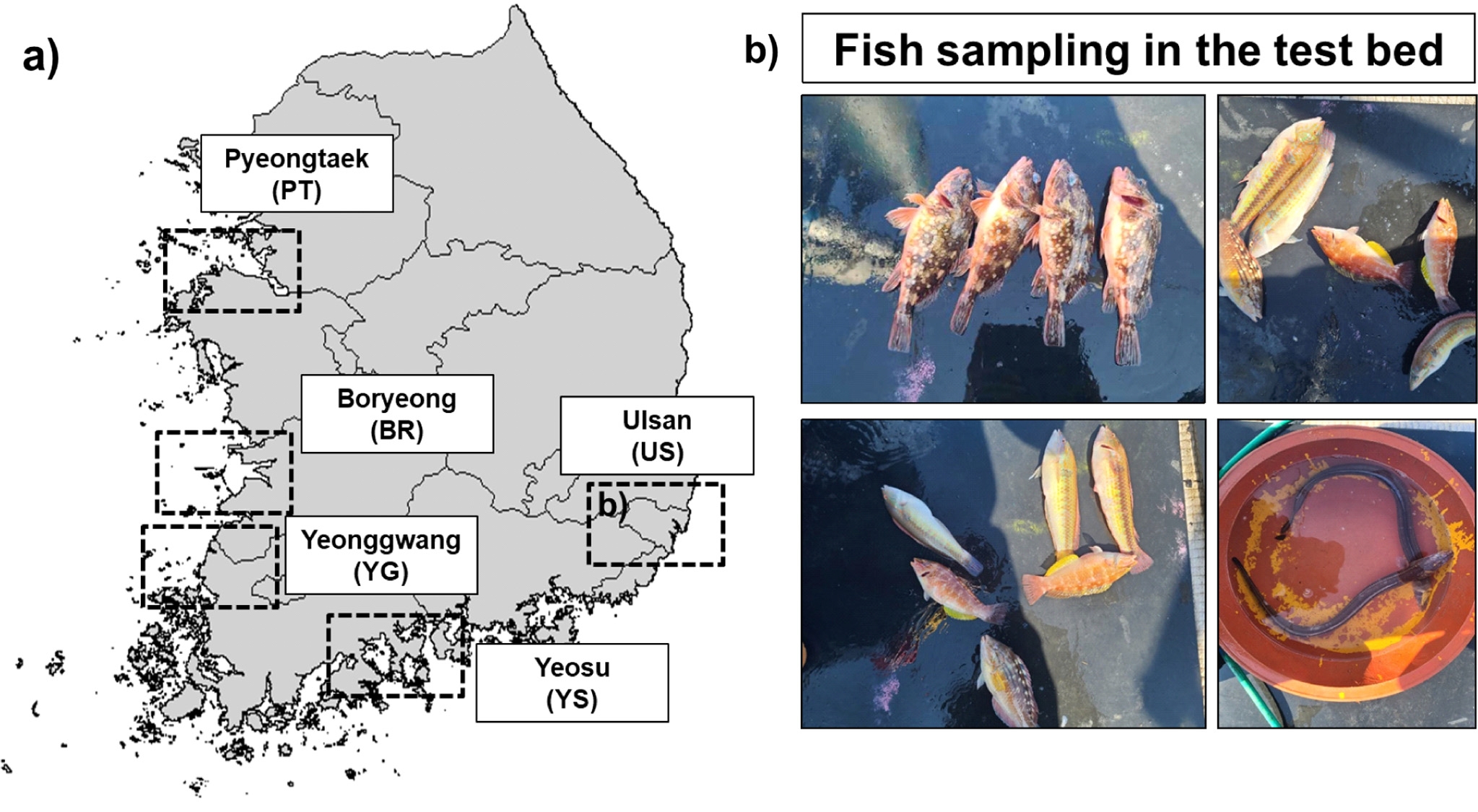
Sampling information for a) the analysis of isotopic compositions (carbon and nitrogen) in particulate organic matter (POM) and b) marine organisms (fish and sea eel) collected at the test-bed (Onsan bay, Ulsan).
2.2 탄소 및 질소 안정동위원소 분석
채취된 시료(입자성 부유물질(particulate organic matter, POM), 동물플랑크톤, 어류))의 안정동위원소(탄소, 질소) 분석을 위해 동결건조하였다. POM의 경우, 동결건조 후 데시케이터를 이용하여 1N 염산 증기로 무기탄소를 제거하였다. 동물플랑크톤의 경우 탄소 및 질소 안정동위원소비(δ13C 및 δ15N) 측정을 위해 분쇄하여 균질화를 진행하였다. 어류의 경우, 근육조직을 사용하였으며 분말형태로 균질화 후 지질제거(Dichloromethane : Methanol, 99 : 1, v/v)과정을 수행하였다. 전처리가 완료된 시료들은 안정동위원소 분석을 위해 시료의 무게를 측정하여 주석(8 mm × 5 mm, Isoprime, UK)에 담아 밀봉한 후 원소분석기(elemental analyzer, Elementar, Germany)-동위원소질량분석기(isotope ratio mass spectrometry, GV instrument, UK)를 이용하여 측정하였다. 안정동위원소 비는 ‰로 표현하며, δ계산식은 아래와 같다.
(1) |
- where R: 13C/12C, 15N/14N, respectively
측정값은 탄소, 질소 각각 VPDB(Vienna Pee Dee Belemnite), 대기 중 N2에 대한 천분율(permil: ‰) 값으로 환산하여 계산에 이용하였다. δ13C와 δ15N 분석에 사용되는 표준가스(CO2 gas: 99.999%, N2 gas: 99.999%)의 델타(δ) 값 보정을 위해 표준물질(IAEA-CH-3: -24.7‰ 및 IAEA-N-1: 0.4‰)이 사용되었으며, δ13C 및 δ15N 분석 정밀도는 각각 ±0.2‰ 이하로 측정되었다.
2.3 아미노산 안정동위원소 분석
아미노산의 δ15N 분석을 위하여 균질화된 어류시료는 기존에 정립된 분석방법에 따라 전처리를 진행하였다(Chikaraishi et al.[2007]). 6 M 염산을 이용하여 균질화된 건시료를 110℃에서 12시간 동안 가수분해를 진행하였다. 시료내 소수성 물질(지질) 제거를 위해 n-Hexane : Dichlormethane(3 : 2, v/v)를 사용한 후, 유도체 반응을 진행하였다. 유도체 반응은 Thionyl chloride : 2-Propanol(1 : 4, v/v)와 Pivaloyl chloride : Dichloromethane(1 : 4, v/v)를 각각 이용하였으며, 1차적으로 110℃에서 2시간 진행 후 추가적으로 동일온도에서 2시간 동안 진행되었다. 유도체 반응이 끝난 아미노산 분자는 n-Hexane : Dichlormethane(3 : 2, v/v)를 이용하여 추출하였다. 전처리가 끝난 시료는 가스크로마토그래피(gas chromatography, HP 6890N, Agilent, USA)-안정동위원소 질량분석기(isotope ratio mass spectrometry, Isoprime 100, UK)를 이용하여 δ15NAAs를 분석하였다. 분석의 정확성을 확인하기 위해 12종의 아미노산 표준물질(alanine, glycine, valine, leucine, isoleucine, proline, aspartic acid, threonine, serine, methionine, glutamic acid, phenylalanine)을 시료 분석 5~7회마다 분석하였다. 표준물질의 δ15NAAs값을 이용하여 측정시료의 δ15NAAs값을 보정하였으며, δ15N 분석 정밀도는 ±0.7‰ 이하로 측정되었다.
3. 결과 및 고찰
3.1 해양산업시설 주변 안정동위원소 분포 및 특성
해양산업시설 주변에서 채취한 POM의 δ13C와 δ15N 값은 지역에 따라 다양한 범위(울산; -23.9±1.1‰ 및 0.9±3.0‰, 여수; -22.2±1.0‰ 및 5.8±1.1‰, 영광; -20.3±0.6‰ 및 4.8±0.9‰, 보령; -21.6±0.6‰ 및 2.7±0.5‰, 평택; -24.7±1.7‰ 및 11.8±1.2‰)를 보였다(Fig. 2). 일반적으로 δ13C의 경우, 대부분 12C와 13C의 형태로 존재하며 자연환경 내 넓은 범위를 포함한다(Craig, 1957; Fry, 1988). 이와 관련하여 육상기원 C3계열 식물의 δ13C값은 –35‰~–0‰의 범위를 보이며, 해양생물(식물플랑크톤)의 δ13C값은 상대적으로 무거운 동위원소비(–0‰~–8‰)를 보인다(Smith and Epstein[1971]; Heckathorn et al.[1999]; Rau et al.[1982]). 더욱이 석유기원 유기물의 δ13C값은 –9‰~–0‰의 범위를 보이며(Calder and Parker[1968]), 과거 동화/이화작용 동안 퇴적유기물의 기원 차이에 따라 차별적인 동위원소 범위를 보인다(Chung et al.[1992]). 한편, δ15N는 대부분 14N와 15N의 형태로 존재한다(Mariotti[1983]). 대기 중 δ15N는 0‰이며, 식물에 의한 생물학적 질소 고정(biological N2 fixation) 과정 동안 동위원소 분별은 매우 작아서 δ15N가 0‰에 가깝다(Fry[1988]). 더욱이, 육상식물의 δ15N값(−1~4‰)은 해양기원 유기물(3-12‰)보다 가벼운 값을 가진다고 알려져 있다(Peters et al.[1978]; Ehleringer and Rundel[1989]; Hedges et al.[1997]; Cloern et al.[2002]). 인공 암모니아 합성비료 내 δ15N값은 −5.9‰까지 가벼운 범위를 보이며(Bateman and Kelly[2012]), 탈질신화 과정에 의해 나타나는 δ15N값(8-16‰)과는 차별적인 특징을 보인다(Bateman and Kelly[2012]). 이를 기반으로, 본 연구에서 POM 내 지역간 차별적인 δ13C와 δ15N 값을 확인하였으며, 영광에서 상대적으로 무거운 δ13C값(−20.3±0.6‰)은 해양산업시설 주변 내 우점적인 식물플랑크톤 기원을 반영하는 것으로 보인다. 전국 연안환경 내 엽록소 농도가 서해 남부에서 전반적으로 높게 나타나고 있음을 고려할 때(6.7±3.8 ug/L; Lee et al.[2022]), 영광 주변 높은 식물플랑크톤 성장은 지역간 차별적인 해양환경특성(온도, 영양염, 빛, 성층화)에 의해 영향을 받을 것이라 판단된다. 한편, 평택에서 나타난 δ13C(−24.7±1.7‰)와 δ15N(11.8±1.2‰) 값은 외부기인 유기물의 유입(예; 육상식물 및 인공합성비료) 증거를 분명히 반영하며, 그 외 지역(울산, 여수, 보령)에서는 POM 내 δ13C와 δ15N 값을 통해 자생 및 외부기원 유기물의 혼합기여를 반영할 것으로 판단된다.

Spatial variation of carbon (a), and nitrogen (b) isotopic compositions (δ13C and δ15N) of POM for five marine industrial facilities (US; Ulsan, YS; Yeosu, YG; Yeonggwang, BR; Boryeong, PT; Pyeongtaek). Each dash line (I-V) indicates the representative end-members within Korean coasts (Algae, petroleum, C3 plant, denitrification, synthetic nitrogen fertilizer).
3.2 HNS 생물축적 평가를 위한 안정동위원소 적용 필요성
현재까지 HNS 관리 우선순위 선정을 위해 국내 화학물질 우선순위 관리기법(CRS-KOREA II)를 기반으로, 선정 목적에 따라 세부 평가지표들을 일부 변경하였다(Hansen et al.[1999]; Choi et al.[2005]; Park et al.[2005]; Neuparth et al.[2011]; Harold et al.[2014]). 해상 HNS 관리 우선순위 선정시스템 내 최종 위해도는 인체와 수생태 위해성의 합(Riskhuman+Riskaquatic)의 관계로 설정하여 평가하고, 각 위해성 지표는 독성(toxicity)과 노출지표(exporsure)의 곱의 관계로 설정하였다(Kim et al.[2015]). 수생태계 위해성 평가의 경우, (1)수생생물 영양단계별 급성독성, 만성독성값을 통해 독성지표를 선정한 바 있으며, (2)생태노출지표는 해상운송량(톤/년), 잔류성, 축적성, 인화점, 발화점, 수용해도를 포함하여 종합적인 DB구축 및 점수화를 진행시킨바 있다(Kim et al.[2015]). 하지만 위해성 정보에 관한 각 지표들의 점수화 결과, 약 40% 정도의 측정치만이 확보되었으며, 인체 아/만성독성, 기타독성, 수생태 독성/생물 축적 등과 같은 지표의 결과치 확보는 현재까지 미비한 단계이다. 특히 수생태 독성의 경우, 해양생물종을 대상으로 한 자료는 극히 부족한 실정이며 체계적인 해상 HNS 사고 관리를 위해서 현장기반의 HNS 해양생물 축적 자료 확보가 필요할 것이라 판단된다.
해양 생태계 내 주요 생물의 먹이사슬 구조 평가와 관련하여, δ13C와 δ15N 값은 서식생물의 주된 먹이원 및 영양단계 평가에 활용되어 왔다(DeNiro and Epstein[1981]; Minagawa and Wada[1984]). 우선적으로 위의 기법을 적용하기 위해, 해양 생태계 내 서식하는 생물종 뿐만 아니라 이들의 에너지원으로 추정되는 먹이원(수생식물, 퇴적유기물, 부유성 입자유기물질)을 함께 채집함으로서 배경질소값(용존무기질소) 변화에 따른 1차 생산자의 δ15N값 변동성 파악이 필요하다(Fry[1991]; Vander Zanden and Rasmussen[1999a]; Vander zanden et al.[1999b]; Dubois et al.[2007]; Gal et al.[2012]). 특히, 먹이사슬 구조(먹이원-상위섭식자) 내 일정하게 발생되는 δ15N 분별작용(fractionation) 원리를 활용하여 생물간 생태계 지위를 체계적으로 파악할 수 있으며(Fry and Sherr[1989]; Fry[1991]), 이를 토대로 다양한 환경 요인(예; 먹이생물의 자원량, 생태계 크기, 교란작용)에 의한 영양단계 변동을 효과적으로 진단 할 수 있다(Post[2002]; Doi et al.[2009]; Mchugh et al.[2010]). 먹이원과 섭식자의 δ15N값 차이는 영양단계에 따른 안정동위원소 분별 계수(trophic discrimination factor, TDF)로 정의되며 다음 식을 활용하여 생물 영양단계를 산출 할 수 있다.
(2) |
- where con: consumer, pro: producer
현재까지 보편적으로 사용되는 TDF는 3.4±1.1‰로 알려져 있으나(Minagawa and Wada[1984]), 다양한 환경조건 내 생물 영양단계 평가에 대한 오류가 보고되고 있는 실정이다(Rolff[2000]; Hannides et al.[2009]). 실제로 TDF는 생물 종 간(Vander zanden and Rasmussen[1999a]; Post[2002]; Dubois et al.[2007]), 생체 시료의 부위별(Reich et al.[2008]), 먹이질에 따라 동위원소 분별 차이가 발생될 수 있다(Robbins et al.[2005]). 실제로 수생태계 1차 생산자의 δ15N 값의 시공간적 변화에 따라 상위 영양단계 생물의 생태계 지위 변동 가능성을 국외사례를 통해 확인되어진 바 있다(Rolff[2000]; Hannides et al.[2009]). 국내의 경우도 자생(부착조류) 및 외부기원(육상 유기오염)에 따른 POM의 δ15N값 변동성으로 인해 안정동위원소 기반 영양단계 평가의 제한점을 도출한 바 있다(Kim et al.[2014]; Kim et al.[2022]). 이를 보완하기 위해 추가적인 연구분석 방법(분자수준 동위원소 분석, compound-specific isotopic analysis)에 대한 필요성 제기되고 있는 실정이다.
복잡한 수생태계 환경 내 서식생물 사이에 정확한 영양단계 산출을 위해 아미노산(amino acid)의 δ15N 분석기법이 최근 활발히 활용되고 있다(Mccllend and Montoya[2002]; Chikaraishi et al.[2007]; McMarthy et al.[2007]; Ishikawa et al.[2014]; Choi and Shin[2018]). 이와 관련하여 영양단계에 따라 δ15N값이 변동되지 않는 아미노산(페닐알라닌) 및 변동될 수 있는 아미노산(글루타민산)이 확인되었으며(McMarthy et al.[2007]), 생물 체내에서 각 아미노산의 대사과정 차이에 의해 나타날 수 있는 δ15N의 분별특성을 활용하여 보다 신뢰성 있는 서식생물의 영양단계 산출 가능성을 확인하였다. 이를 기반으로 단일 생물 섭식자 내 페닐알라닌과 글루타민산의 δ15N값을 활용한 영양단계 산출식은 다음과 같다.
(3) |
위 식에서 β는 기초생산자의 페닐알라닌과 글루타민산의 δ15N값(δ15Nphe, δ15Nglu) 차이(3.4‰)로 표현되며, TDF는 7.6‰이 사용되고 있다(Chikaraishi et al.[2007]). 아미노산 동위원소 분석 방법은 하나의 생물 체내에서 분석된 δ15Nphe와 δ15Nglu값을 통해 먹이원 및 영양단계 정보를 동시에 확인할 수 있으며, 다양한 먹이원의 δ15N값 변동성으로 인한 상위 생태계 지위평가의 불확실성을 보완할 수 있다(Chikaraishi et al.[2010]; Choi and Shin[2018]; Won et al.[2018]; Kim et al.[2022]). 특히 본 연구에서 평가된 해양산업시설 주변 해역 간 확인된 POM의 δ15N값 변동성은 기존 총 조직(bulk tissue) 내 δ15N값을 활용한 영양단계 산출시, 동일 해양생물 종에 대한 영양단계가 지역별 서로 다른 생태계 지위를 가질 수 있음을 의미한다. 따라서, 해양산업시설 주변 해역 내 기초생산자에 대한 질소원 변화가 큰 환경조건에서 아미노산의 δ15N 분석기법을 활용함으로서 보다 신뢰성 있는 영양단계 산출이 가능할 것으로 판단된다. 더욱이 전국적으로 분포하는 해양산업시설 주변 HNS 생물 축적에 관한 통합적인 평가와 관련하여, 외부영향으로 인한 배경 δ15N 값이 시공간적으로 영향을 받지 않는 독립적인 평가방법이 고려되어야 할 것으로 판단된다.
3.3 테스트배드 내 서식생물의 영양단계 및 HNS 생물 축적 평가방안
HNS의 생물축적성 평가를 위해 우선적으로 단일 테스트베드(울산 온산만) 내 POM, 동물플랑크톤(4종), 어류(5종)를 채집하였으며, 분석된 δ13C와 δ15N값은 시료간 일정한 동위원소 범위(POM; −22.8±0.8‰ 및 4.7±1.8‰, 동물플랑크톤; −20.7±1.1‰ 및 8.7±1.3‰, 어류; −15.6±0.4‰ 및 13.0±0.3‰)차이를 보였다(Fig. 3a). 동물플랑크톤의 δ13C와 δ15N값의 경우, 우점종(Parasagitta elegans, Euchaeta plana, Paracalanus parvus S.I, Calanus sinicus.)들 사이에 최대 δ15N값 차이(~3‰)를 보이며 이는 테스트베드 내 종들간 먹이원 및 에너지 흐름 변화와 관련 있을 것으로 판단된다(Fry and Sherr[1989]; Lee et al.[2008]; Kim et al.[2014]). 본 연구에서 분석한 요각류 4종 중 P. parvus s. l.과 C. sinicus는 각각 나노 크기(2-20 μm)와 마이크로 크기(20-200 μm)의 POM를 주로 섭취하는 여과식자로 알려져 있으며(Im et al.[2015]; Benedetti et al.[2016]), Euchaeta plana와 Parasagitta elegans는 소형 요각류를 주로 섭취하는 것으로 알려져 있다(Alvarez-Cadena[1993]; Benedetti et al.[2016]). 이를 고려할 때, 동물플랑크톤 종(초식성 및 육식성)간의 δ15N 동위원소 변동성은 이들의 먹이 섭식 특성을 분명히 반영한 결과라 판단된다. 테스트베드 내 채집된 주요 어류종은 대부분 저서환경에서 서식하는 것으로 알려져 있으며(http://www.fishbase.in), 이들의 δ13C와 δ15N값은 POM과 동물플랑크톤의 동위원소 값에 비해 유의미한 농축 경향(p<0.01)을 보였으나, 어류종 간에 뚜렷한 동위원소 차이는 확인할 수 없었다(Fig. 3). 어류의 δ13C값은 수층 POM δ13C값에 비해 최대 4‰ 무거워짐에 따라, 어류의 탄소원은 표영성(pelagic) 동물플랑크톤 뿐만 아니라 저서성(benthic) 유기체 섭식 영향과 관련 있을 것으로 판단된다(Kim et al.[2012]). 더욱이 측정된 배경질소원-동물플랑크톤의 δ15N값을 기반으로 추정되는 어류의 δ15N값(~16‰)을 고려했을 때, 가볍게 측정된 어류종의 δ15N값(12.7±0.1‰)은 저서성 유기체 섭식 에 대한 잠재적 증거를 반영한 것이라 판단된다.
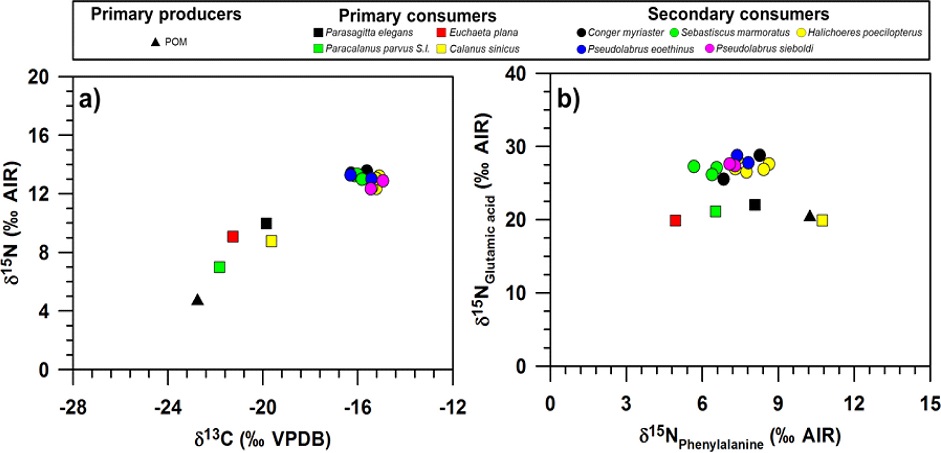
Dual plots of isotopic compositions in bulk elements (a; δ13C and δ15N) and specific amino acids (b; δ15Npheny lalanine and δ15NGlutamic acid).
테스트베드 내 채취된 시료 내 측정된 δ15Nphe, δ15Nglu값은 서로 다른 동위원소 범위(POM; 10.3±5.2‰ 및 20.3±9.2‰, 동물플랑크톤; 8.0±2.2‰ 및 20.9±1.2‰, 어류; 7.6±1.2‰ 및 27.5±1.0‰)를 보였다(Fig. 3b). 일반적으로 수생물 내 페닐알라닌의 δ15N값은 먹이공급원 정보를 제공할 수 있다는 점을 고려했을 때(Kim et al.[2022]; Won et al.[2018]), 다른 동물플랑크톤 종에 비해(δ15Nphe; 6.7±1.4‰), C. sinicus은 δ15Nphe값이 상대적으로 가장 무겁게 나타났다. P. parvus s. l.이 주로 나노 크기의 POM을 주로 섭취하며, E. plana, P. elegans가 주로 P. parvus s. l.을 비롯한 소형 요각류를 섭식하는 반면, C. sinicus는 주로 마이크로 크기의 POM을 섭취한다는 점에서 서로 다른 특징을 보인다(Alvarez-Cadena[1993]; Im et al.[2015]; Benedetti et al.[2015]). 따라서, 본 연구에서 발견한 동물플랑크톤 종 사이에 확인된 δ15Nphe의 변동성은 다양한 생물학적 차이(예; 먹이의 농도, 먹이 입자들의 크기/질)에 의해 영향을 받는 것으로 보인다. 한편, 어류에서 측정된 δ15Nphe의 경우 대부분 유사한 동위원소 범위를 보이고 있으며(Fig. 3), 이는 테스트베드 내 서식하는 어류의 먹이원은 대부분 유사한 것으로 판단된다. 더욱이 동물플랑크톤의 δ15Nglu값(20.9±1.2‰)에 비해, 어류에서 측정된 δ15Nglu값은 일정한 농축값(27.5±1.0‰)을 보였으며 전형적인 TDF값(~8‰)을 반영한 상위영양단계 특징을 보였다. 이와 관련하여 아미노산 기반 영양단계 산출식(2)에 사용되는 TDF의 경우, 기존 영양단계 산출식(1)에 비해 크기 때문에 TDF 변동에 의한 영양단계의 오차범위(<±0.2)는 줄어들 수 있다고 보고된바 있다(Bowes and Thorp[2015]). 또한 다양한 생물학적 조건(예; 생체부위, 대사작용)에서도 TDF 변동차이가 없음이 규명됨에 따라(Chikaraishi et al.[2014]), 이를 기반으로 해양산업시설 주변해역 내 주요 해양생물 종에 대한 체계적인 영양단계를 추정할 수 있을 것이라 판단된다.
테스트베드 내 안정동위원소를 활용하여 산출된 영양단계(trophic position, TP)는 표 1에 나타냈다. 우선적으로 식 (1)과 (2)으로 산출된 TP는 양의 상관관계(R2= 0.805, p < 0.05)를 나타냈으며(Fig. 4), 소형크기 요각류 섭식 특성을 가지는 P. parvus s. l.종의 영양단계(TPBulk-TPAA; −0.8±0.1) 차이는 가장 큰 변화를 보였다. 이와 관련하여, 외부기인 환경 영향에 따라 배경 δ15N값이 변동될 수 있음을 고려했을 때(Choi and Shin[2018]), 다양한 환경요인(현장수온, 원생생물 생체량)으로 영향을 받을 수 있는 동물플랑크톤의 TPbulk는 변동될 수 있을 것으로 보인다(TPBulk-TPAA; −0.1±0.6). 더욱이 상위영양단계(TP>3)로 갈수록 어류생물종의 가벼워지는 δ15N값 특성(TPBulk-TPAA; −0.4±0.1)은 단일 TPbulk의 적용시 테스트베드 내 일부 서식생물종 사이에 과소평가된 TP를 나타낼 수 있을 것이라 판단된다. 이를 토대로, 본 연구에서 산출된 TP 평가는 글로벌 TP 데이터베이스(http://www.fishbase.in)에 나타난 각 어류종의 TP값과 비교를 하였다(Fig. 4). ΔTP값은 TPbulk-fishbase(−0.1±0.3)와 TPAA-fishbase(0.3±0.4)로 각각 표현되었으며, 식 (1)과 (2)로 산출된 TP값과 비교했을 때 통계적 유의한 차이(p > 0.01)는 보이지 않았다. 따라서, 테스트베드 내 수행된 생물 영양단계 방법은 일부 생물종에서 외부 환경요인으로 인한 차별적인 δ15N 변동성이 반영될 수 있지만, 테스트베드 내 두 방법 모두 현장기반 HNS 생물축적 평가에 적용 가능할 것으로 예상된다. 추후, 전국단위 수준에서 해양산업시설 주변 해양생물종간 TP 변동자료를 확보하여 보다 신뢰성 있는 HNS 생물축적 평가를 진행해야 할 것이라 판단된다.

Comparison for trophic positions via (a) the analysis of bulk isotopes and amino acids, and (b) TPAA-Fishbase and TPBulk-Fishbase. Each color symbol (triangle, square, and circle) was mentioned in Figure 3. Alphabet letter indicates a statistically non-significant group of data (p > 0.01) determined using analysis of variance and post-hoc Duncan test.
HNS의 생물축적성 평가를 위해, 현장기반 해양생물 종간 영양단계 및 이들 생물 내 특정 HNS의 농도 사이에 선형회귀관계를 분석하여 다음과 같은 식을 이용하여 먹이망확대계수(trophic magnification factor, TMF)를 도출할 수 있다(Borga et al.[2012]).
(4) |
(5) |
본 연구에서 평가된 TP값을 활용하여 계산된 TMF는 각 생물 종(예; 표영성, 저서성)에 따른 영양단계에 기반한 체내 HNS의 축적 정도를 효과적으로 파악할 수 있을 것이라 판단된다. 실제로 수생태계 내 다양한 유해물질(synthetic musk compounds, siloxanes, mercury, persistent organic pollutants)의 생물축적 평가를 위해 안정동위원소 기반 TP값을 활용하여 다양한 생물종 사이의 TMF 변화를 효과적으로 규명한 바 있다(Kim et al.[2012]; Kim et al.[2022]; Won et al.[2020]). 더욱이, 먹이생태계 내 TMF의 변동성은 화학물질의 물리화학적 특성(pysicochemical properties) 차이(예; 소수성/친수성, 끓는점, 증기압 등)로 인해 영향을 받을 수 있으며(Borga et al.[2012]; Conder et al.[2008]), 추후 HNS의 생물축적 평가에 있어서 TMF와 함께 중요한 조건인자로서 필수적인 고려가 필요할 것이라 판단된다. 현재까지 해양환경관리법상에 등재되어 있는 대부분의 HNS는 주로 분자량이 낮고, 휘발성이 강한 물질위주로 구성되어 있다. 이들 물질은 낮은 옥타놀-물 분배계수(octanol-water partition coefficient, Kow)를 가지고 있으며, 수생태계 내에서 생물체 내의 지방 등에 축적될 확률이 매우 낮아 TMF를 파악하기 어려움이 있을 것으로 예상된다(Kelly et al., 2007). 그러나 새롭게 추가될 HNS 후보물질에는 생물축적성에 기반한 생태계의 영향을 고려하여 선정한다면 본 연구에서 제안하는 안정동위원소에 기반한 생물 축적성평가를 통해 HNS 후보물질군을 더욱 효과적으로 제안할 수 있을것이라 판단한다.
4. 결 론
본 연구에서는 해양사업시설 주변 HNS의 생물축적평가와 관련하여, 안정동위원소 기반의 해양생태계 생물영양단계 평가를 우선적으로 수행하였다. 테스트베드 내 채집된 해양생물종의 총 조직 내 안정동위원소(δ13C, δ15N) 및 아미노산(δ15Nphe, δ15Nglu) 분석을 통해 이들의 영양단계 특성을 비교·검증하였다. 적어도 테스트베드 내 두 방법을 사용한 TP 평가는 현장기반 HNS 생물축적성 규명을 위해 모두 적용 가능할 것으로 예상되나, 추가적인 생물자료 확보를 통해 TP평가 신뢰성을 향상시킬 필요가 있을것으로 판단된다. 더욱이 HNS 위해성 평가시, 현장기반의 수생태계 독성자료가 미비한 점을 고려했을 때 본 연구에서 확립한 영양단계 자료는 추후 다양한 생물종 사이에 TMF값을 도출하는데 유용한 기초자료로서 활용될 수 있을 것이라 판단된다. 우선관리 대상으로 선정된 HNS 및 이들의 방출량을 비교함으로써 더욱 신뢰성 있는 현장기반 TMF값을 도출할 수 있을 것이라 판단된다. 이를 기반으로 전국단위 해양산업시설 주변 HNS별 생물농축 데이터베이스 구축 및 생태 유해성 평가기술의 고도화를 통해 체계적인 HNS 배출허용기준이 결정됨으로서 국가관리정책 수립에 중요한 정책의사 자료를 제공할 수 있을 것이라 판단된다.
Acknowledgments
이 논문은 2022년도 해양수산부 재원으로 해양수산과학기술진흥원의 지원을 받아 수행된 연구임(20210660, 해양산업시설 배출 위험유해물질 영향평가 및 관리기술 개발).
References
-
Alvarez-Cadena, J.N., 1993, Feding of te Chaetognath Sagitta elegans Verrill,, Estuar. Coast. Shelf Sci, 36(2), 195-206.
[https://doi.org/10.1006/ecss.1993.1013]
-
Bateman, A. and Kelly, S.D., 2012, Fertilizer notrogen isotope signatures, Isot. Environ. Health Stud, 43, 237-247.
[https://doi.org/10.1080/10256010701550732]
-
Benedetti, F., Gasparini, S. and Ayata, S.D., 2016, Identifying copepod functional groups from species functional traits, J. Plankton Res, 38(1), 159-166.
[https://doi.org/10.1093/plankt/fbv096]
-
Borga, K., Fjeld, E., Kierkegaard, A. and McLachlan, M.S., 2012, Food Web Accumulation of Cyclic Siloxanes in Lake Mjosa. Norwa. Environ. Sci. Technol, 46, 6347-6354.
[https://doi.org/10.1021/es300875d]
-
Bowes, R.E. and Thorp, J.H., 2015, Consequences of Employin Amino Acid vs. Bulk-Tissue, Stable Isotope Analysis: A Laboratory Trophic Position Experiment, Ecosphere, 6, 1-12.
[https://doi.org/10.1890/ES14-00423.1]
-
Calder, J. and Parker, P.L., 1968, Stable carbon isotope ratios as indexes of petrochemical pollution of aquatic systems, Environ. Sci. Technol, 2, 535-539.
[https://doi.org/10.1021/es60019a001]
-
Chikaraishi, Y., Kashiyama, Y., Ogawa, N.O., Kitazato, H. and Ohkouchi, N., 2007, Metabolic Control of Nitrogen Isotope Composition of Amino Acids in Macroalgae and Gastropods: Implications for Aquatic Food Web Studies, Mar. Ecol. Prog. Ser, 342, 85-90.
[https://doi.org/10.3354/meps342085]
- Chikaraishi, Y., Ogawa, N.O. and Ohkouchi, N., 2010, Further Evaluation of the Trophic Level Estimation Based on Nitrogen Isotopic Composition of Amino Acids, 37-51, In: Earth, ife, and isotopes, Kyoto Univ. Press.
-
Chikaraishi, Y., Steffan, S.A., Ogawa, N.O., Ishikawa, N.F., Sasaki, Y., Tsuchiya, M. and Ohkouchi, N., 2014, High-Resolution Food Webs based on Nitrogen Isotopic Composition of Amino Acids, Ecol. Evol, 4, 2423-2449.
[https://doi.org/10.1002/ece3.1103]
-
Cho, S.J., Kim, D.J. and Choi, K.S., 2013, Hazardous and Noxious Substances (HNS) Risk Assessment and Accident Prevention Measures on Domestic Marine Transportation, J. Korean Soc. Mar. Environ, 19, 145-154.
[https://doi.org/10.7837/kosomes.2013.19.2.145]
- Choi, S.P., Park, H.S., Lee, D.S., Shin, Y.S., Kim, Y.S. and Shin, D.C., 2005, Development of CRS-Korea II and its Application to Setting the Priority of Toxic Chemicals for local Provinces, Environ. Toxicol, 20, 311-325.
- Choi, B. and Shin, K.H., 2018, Applications and Prospects of Stable Isotope in Aquatic Ecology and Environmental Study, Korean J. Environ. Ecol, 47, 53-61.
-
Cloern, J.E., Canuel, E.A. and Harris, D., 2002, Stable Carbon and Nitrogen Isotope Composition of Aquatic and Terrestrial Plants of the San Francisco Bay Estuarine System, Limnol. Oceanogr, 47, 713-729.
[https://doi.org/10.4319/lo.2002.47.3.0713]
-
Conder, J.M., Hoke, R.A., Wolf, W.D., Russell, M.H. and Buck, R.C., 2008, Are PFCAs Bioaccumulative? A Critical Review and Comparison with Regulatory Criteria and Persistent Lipophilic Compounds, Environ. Sci. Technol, 42, 995-1003.
[https://doi.org/10.1021/es070895g]
-
Craig, H., 1957, Isotopic Standards for Carbon and Oxygen and Correction Factors for Mass-Spectrometric Analysis of Carbon Dioxide, Geochim. Cosmochim. Acta, 12, 133-149.
[https://doi.org/10.1016/0016-7037(57)90024-8]
-
DeNiro, M.J. and Epstein, S., 1981, Influence of Diet on the Distribution of Nitrogen Isotopes in Animals, Geochim. Cosmochim. Acta, 45, 341-351.
[https://doi.org/10.1016/0016-7037(81)90244-1]
-
Doi, H., Chang, K.H., Ando, T., Ninomiya, I., Imai, H. and Nakano, S.I., 2009, Resource Availability and Ecosystem Size Predict Food-Chain Length in Pond Ecosystems, Oikos, 118, 138-144.
[https://doi.org/10.1111/j.1600-0706.2008.17171.x]
-
Dubois, S., Jean-Louis, B., Bertrand, B. and Lefebvre, S., 2007, Isotope Trophic-Step Fractionation of Suspension-Feeding Species: Implications for Food Partitioning in Coastal Ecosystems, J. Exp. Mar. Biol. Ecol. 351, 121-128.
[https://doi.org/10.1016/j.jembe.2007.06.020]
-
Ehleringer, J.R and Rundel, P.W., 1989, Stable Isotopes: History, Units, and Instrumentation, 1-15. In: Stable isotopes in ecological research. Springer, New York, NY.
[https://doi.org/10.1007/978-1-4612-3498-2_1]
-
Fry, B, 1988, Food Web Structure on Georges Bank from Stable C, N, and S Isotopic Compositions, Limnol. Oceanogr, 33, 1182-1190.
[https://doi.org/10.4319/lo.1988.33.5.1182]
-
Fry, B. and Sherr, E.B., 1989, δ13C Measurements as Indicators of Carbon Flow in Marine and Freshwater Ecosystems, In Stable Isotopes in Ecological Research, Springer, 196-229.
[https://doi.org/10.1007/978-1-4612-3498-2_12]
-
Fry, B., 1991, Stable Isotope Diagrams of Freshwater Food webs, Ecology, 72, 2293-2297.
[https://doi.org/10.2307/1941580]
- Gal, J.K., Kim, M.S., Lee, Y.J., Seo, J. and Shin, K.H., 2012, Foodweb of Aquatic Ecosystem within the Tamjin River through the Determination of Carbon and Nitrogen Stable Isotope Ratios, Korean J. Environ. Ecol, 45, 242-251.
-
Hannides, C.C., Popp, B.N., Landry, M.R. and Graham, B.S., 2009, Quantification of Zooplankton Trophic Position in the North Pacific Subtropical Gyre using Stable Nitrogen Isotopes, Limnol. Oceanogr, 54, 50-61.
[https://doi.org/10.4319/lo.2009.54.1.0050]
-
Hansen, B.G., Haelst, A.G.V., Leeuwen, K.V. and Zandt, P.V.D., 1999, Priority Setting for Existing Chemicals: European Union Risk Ranking Method, Environ. Toxicol. Chem, 18, 72-779.
[https://doi.org/10.1002/etc.5620180425]
-
Harold, P.D., de Souza, A.S., Louchart, P., Russell, D. and Brunt, H., 2014, Development of a risk-based prioritization methodology to inform Public health emergency planning and preparedness in case of accidental spill at sea of hazardous and noxious substances (HNS), Environ. Int, 72, 157-163.
[https://doi.org/10.1016/j.envint.2014.05.012]
-
Heckathorn, S.A., McNaughton, S.J., Coleman, J.S., Sage, R.F. and Monson, R.K., 1999, C4 plants and Herbivory, C4 plant biology. 285-312.
[https://doi.org/10.1016/B978-012614440-6/50010-0]
-
Hedges, J.I., Keil, R.G. and Benner, R., 1997, What Happens to Terrestrial Organic Matter in the Ocean?, Org. Geochem, 27, 195-212.
[https://doi.org/10.1016/S0146-6380(97)00066-1]
-
Im, D.H., Wi, J.H. and Suh, S.L., 2015, Evidence for ontogenetic feeding strategies in four calanoid copepods in the East Sea (Japan Sea) in summer, revealed by stable isotope analysis, Ocean Sci. J, 50(3), 481-490.
[https://doi.org/10.1007/s12601-015-0044-y]
- IMO, 2000, Protocol on Preparedness, Response and Cooperation to Pollution Incidents by Hazardous and NoxiousSubstances (OPRC-HNS protocol), Attachment 1, 1-11.
-
Ishikawa, N.F., Kato, Y., Togashi, H., Yoshimura, M., Yoshimizu, C., Okuda, N. and Tayasu, I., 2014, Stable Nitrogen Isotopic Composition of Amino Acids reveals Food Web Struture in Stream Ecosystems, Oecologia, 175, 911-922.
[https://doi.org/10.1007/s00442-014-2936-4]
-
Kelly, B.C., Ikonomou, M.G., Blair, J.D., Morin, A.E. and Gobas, F.A.P.C., 2007, Food web-specific biomagnification of persistent organic pollutants, Science, 317, 236-239.
[https://doi.org/10.1126/science.1138275]
-
Kim, C.S., Kim, S.H., Lee, W.C. and Lee, D.H., 2022. Spatial variability of water quality and sedimentary organic matter during winter season in coastal aquaculture zone of Korea. Mar. Pollut. Bull, 182, 113991.
[https://doi.org/10.1016/j.marpolbul.2022.113991]
-
Kim, E., Kim, H., Shin, K.H., Kim, M.S., Kun, S.R., Lee, B.G. and Han, S., 2012, Biomagnification of Mercury through the Benthic Food Webs of a Temperate Estuary: Massn Bay, Korea, Environ. Toxicol. Chem, 31, 1254-1263.
[https://doi.org/10.1002/etc.1809]
-
Kim, M.S., Lee, Y.J., An, K.G., Kim, B.H., Hwang, S.J. and Shin, K.H., 2014, Allochthonous Organic Matter Contribution to Foodweb in Shingu Agricultural Researvoir after Rainfall Period, Korean J. Environ. Ecol, 47, 53-61.
[https://doi.org/10.11614/KSL.2014.47.1.053]
-
Kim, Y.R., Kim, T.W., Son, M.H., Oh, S. and Lee, M., 2015, A Study on Prioritization of HNS Management in Korean Waters, J. Korean Soc. Mar. Environ. Saf, 2, 672-678.
[https://doi.org/10.7837/kosomes.2015.21.6.672]
-
Kim, D., Cho, H.E., Won, E.J., Kim, H.J., Lee, S., An, K.G., Moon, H.B. and Shin, K.H., 2022, Environmental Fate and Trophic Transfer of Synthetic Musk Compounds and Siloxanes in Geum River, Korea: Compound-Specific Nitrogen Isotope Analysis of Amino Acids for Accurate Trophic Position Estimation, Environ. Int, 161, 107123.
[https://doi.org/10.1016/j.envint.2022.107123]
- Lee, J.Y., Kim, B.C., Yoshioka, T. and Hino, S., 2008, The Different Isotopic Signatures of Co-existing Zooplankton Species in Two Alpine Lakes, Korean J. Ecology and Environment, 41, 294-300.
-
Lee, M. and Jung, J.Y., 2013, Risk Assessment and National Measure Plan for Oil and HNS Spill Accidents Near Korea, Mar. Pollut. Bull, 73, 339-344.
[https://doi.org/10.1016/j.marpolbul.2013.05.021]
-
Lee, M. and Oh, S., 2014, Development of Response Scenario for a Simulated HNS Spill Incident, J. Korean Soc. Mar. Environ. Saf,, 20, 339-344.
[https://doi.org/10.7837/kosomes.2014.20.6.677]
-
Mariotti, A., 1983, Atmospheric Nitrogen is a Reliable Standard for Natural 15N Abundance Measurements, Nature, 303, 685-687.
[https://doi.org/10.1038/303685a0]
-
McHugh, P.A., McIntosh, A.R. and Jellyman, P.G., 2010, Dual Influences of Ecosystem Size and Disturbance on Food Chain Length in Streams, Ecol. Lett, 13, 881-890.
[https://doi.org/10.1111/j.1461-0248.2010.01484.x]
-
Minagawa, M. and Wada, E., 1984, Stepwise Enrichment of 15N along Food Chains: Further Evidence and the Relation between δ15N and Animal Age, Geochim. Cosmochim. Acta, 48, 1135-1140.
[https://doi.org/10.1016/0016-7037(84)90204-7]
- MPSS, 2015, Ministry of Public Safety and Security, http://www.mpss.go.kr/etc/totalSearch.do, .
-
Neuparth, T., Moreira, S.M., Santos, M.M. and Reis-Henriques, M.A., 2011, Hazardous and Noxious Substances (HNS) in the Marine Environment: Prioritizing HNS that pose major Risk in a European Context, Mar. Pollut. Bull, 62, 21-28.
[https://doi.org/10.1016/j.marpolbul.2010.09.016]
- Park, H.S., Kim, T.S., Lee, D.S., Shin, Y.S., Choi, S.P., Park, S.E., Kim, M.H., Yang, J.Y. and Shin, D.C., 2005, Development of Korean Chemical Ranking and Scoring System (CRS-KOREA) and its Application to Prioritizing National Toxic Chemicals, Environ. Toxicol, 20, 109-121.
-
Peters, K.E., Sweeney, R.E. and Kaplan, I.R., 1978, Correlation of Carbon and Nitrogen Stable Isotope Ratios in Sedimentary Organic Matter, Limnol. Oceanogr, 23, 598-604.
[https://doi.org/10.4319/lo.1978.23.4.0598]
-
Post, D.M., 2002, Using Stable Isotopes to Estimate Trophic Position: Models, Methods, and Assumptions, Ecol, 83, 703-718.
[https://doi.org/10.1890/0012-9658(2002)083[0703:USITET]2.0.CO;2]
-
Reich, K.J., Bjorndal, K.A. and Del Rio, C.M., 2008, Effects of Growth and Tissue Type on the Kinetics of 13C and 15N Incorporation in a Rapidly Growing Ectotherm, Oecologia, 155, 651-663.
[https://doi.org/10.1007/s00442-007-0949-y]
-
Robbins, C.T., Felicetti, L.A. and Sponheimer, M., 2005, The Effect of Dietary Protein Quality on Nitrogen Isotope Discrimination in Mammals and Birds, Oecologia, 144, 534-540.
[https://doi.org/10.1007/s00442-005-0021-8]
-
Rolff, C., 2000, Seasonal Variation in δ13C and δ15N of Size-Fractionated Plankton at a Coastal Station in the Northern Baltic Proper, Mar. Ecol. Prog. Ser, 203, 47-65.
[https://doi.org/10.3354/meps203047]
-
Smith, B.N. and Epstein, S., 1971, Two Categories of 13C/12C Ratios for Higher Plants. Plant Physiol, 47, 380-384.
[https://doi.org/10.1104/pp.47.3.380]
-
Vander Zanden, M. and Rasmussen, J.B., 1999a, Primary Consumer δ13C and δ15N and the Trophic Position of Aquatic Consumers, Ecol, 80, 1395-1404.
[https://doi.org/10.1890/0012-9658(1999)080[1395:PCCANA]2.0.CO;2]
-
Vander Zanden, M., Casselman, J.M. and Rasmussen, J.B., 1999b, Stable Isotope Evidence for the Food web Consequences of Species Invasions in Lakes, Nature, 401, 464-467.
[https://doi.org/10.1038/46762]
-
Won, E.J., Choi, B., Hong, S., Khim, J.S. and Shin, K.H., 2018, Importance of Accurate Trophic Level Determination by Nitrogen Isotope of Amino Acids for Trophic Magnification Studies: A Review. Environ. Pollut, 238, 677-690.
[https://doi.org/10.1016/j.envpol.2018.03.045]
-
Won, E.J., Choi, B., Lee, C.H., Hong, S., Lee, J.H. and Shin, K.H., 2020, Variability of Trophic Magnification Factors as an Effect of Estimated Trophic Position: Application of Compound-Specific Nitrogen Isotope Analysis of Amino Acids. Environ. Int, 135, 105361.
[https://doi.org/10.1016/j.envint.2019.105361]