
COVID-19 팬데믹에 따른 수중 음향환경 변화와 해양 어류 및 무척추동물의 생물학적 영향 고찰
초록
선박 운행, 해양 구조물 건설, 자원 탐사 등 인간 활동으로 발생하는 수중소음이 해양 공해로서 대두되고 있다. COVID-19 팬데믹 이후 국가별 봉쇄 조치에 따라 선박 운행량이 감소하였고 이에 따라 수중 음향환경 또한 변화하였지만, 이와 관련된 생물 영향 연구는 미흡한 실정이다. 본 연구에서는 선행연구 리뷰를 통하여 선박 수중소음에 의한 어류 및 무척추동물의 생물 영향 범위를 산정하고 COVID-19 팬데믹 전후 수중 음향환경 변화와 비교 해석을 하고자 한다. 총 20개의 문헌 리뷰를 통해 선박 수중소음이 어류 14종, 무척추동물 7종에 미치는 영향을 생리, 행동적 영향으로 구분하였다. 그 결과 최소 생물 영향 범위는 어류, 무척추동물 각각에서 101–148 dB re 1 μPa, 118–155 dB re 1 μPa로 나타났으며 초기 발달 단계의 생물이 성체에 비해 수중소음에 더 민감한 것으로 보였다. 총 8개의 문헌 리뷰를 통해 COVID-19 전후 수중 음향환경 변화를 확인한 결과, 6개 국가(캐나다, 미국, 바하마, 독일, 프랑스, 뉴질랜드)에서 음압레벨이 1.2–8.0 dB re 1 μPa 감소하였다. 국내의 경우 2020년 총 선박 입출항 수가 2019년 대비 감소하였으나 2021년 회복세를 보여 선박 수중소음이 일시적으로 감소했을 것으로 사료된다. COVID-19 팬데믹이라는 특수한 상황으로 인해 선박 수중소음이 수중 음향환경에 끼치는 소음 공해 수준을 정량화할 수 있게 되었으며 봉쇄 기간동안 주변소음의 감소는 어류 및 무척추동물의 건강에 긍정적으로 작용했을 것이라 판단된다. 향후 선박 수중소음이 해양생물에 미치는 부정적인 영향을 최소화하기 위해서는 국제 실정에 맞는 관리 방안 마련이 시급하다. 따라서 국내 대표 수산생물 종에 대한 수중소음 영향평가와 서식 생물 보호를 위해 공간관리 체제를 이용한 선박 및 선박 수중소음 제한이 필요할 것으로 보인다.
Abstract
Public concern about the effects of anthropogenic noise such as shipping, resource exploration and construction has steadily increased over the past few decades. Due to the COVID-19 lockdown, ship-based activities around the world have decreased, and as a result, the underwater soundscape has changed. However there is insufficient research on the biological effects in regard to this phenomenom. In this study, we analyzed the reviewed data from the studies on the impact of vessel noise on fish and invertebrates, and changes in underwater soundscape between the pre- and during-COVID-19. Through a total of 20 literature reviews, the effects of vessel noise on 14 fish species and 7 invertebrate species were classified into physiological and behavioral effects. As a result, the minimum biological impact range was found to be 101–148 dB re 1 μPa and 118–155 dB re 1 μPa for fish and invertebrates, respectively. Also results show that the organisms in the early life stage were more sensitive to underwater noise than the adults. Compared to pre-COVID-19, underwater sound pressure levels decreased by about 1.2–8.0 dB re 1 μPa during COVID-19 in six countries (Canada, United States, Bahamas, Germany, France, and New Zealand). In Korea, the total number of ships arriving and departing ports have decreased since March 2020, and it would be inferred that the ambient noise levels have decreased. It is highly likely that the reduction in underwater noise due to COVID-19 has brought a positive effect on living organisms. In order to minimize the harmful effects of underwater noise, management systems suitable for domestic conditions has become of urgency. Therefore, to support this, it is necessary to study the effect of underwater noise on domestic aqatic species and limit ship activities and underwater noise using spatial management system.
Keywords:
COVID-19, Underwater soundscape, Vessel noise, Fish, Invertebrate, Biological impact키워드:
코로나-19, 수중 음향환경, 선박소음, 어류, 무척추동물, 생물영향1. 서 론
2020년 3월 세계보건기구(World Health Organization, WHO)는 COVID-19 팬데믹을 선언했고 세계 곳곳에서 봉쇄 조치가 내려졌다. 일부 주요 항구 폐쇄와 경기 침체로 인해 해상 운송량은 감소하였고 사람들의 이동 및 외출 제한으로 관광, 여행, 레저 등을 목적으로 한 여객 운송이 잠정 중단되었다. 그 결과, 컨테이너선, 유조선, 유람선, 레저 선박 등 다양한 부문의 선박 운항 축소로 인해 해양 환경에 미치는 영향이 변화하였다. 특히 선박 통행 고밀도 지역에서는 COVID-19 이후 부유 퇴적물 감소로 인한 탁도 감소, 연안 수질 향상, 수중소음 감소 등 긍정적인 영향이 관찰되었다(Braga et al.[2020]; Callejas et al.[2021]; Jiang et al.[2022]).
해양에서는 지질활동, 생물활동, 인간활동 등으로 인해 주파수 구간별로 소음이 발생하여 수중 음향환경을 구성한다. 최근 해상풍력발전 건설, 해저탐사, 선박통행, 어업, 등의 인간활동으로 수중소음 공해를 유발하고 있다. 그중 선박은 수중소음에 가장 크게 기여하는데 바다의 주변소음(ambient noise)은 선박으로 인해 1950년부터 10 년당 약 3.3 dB씩 증가하였다(Frisk[2012]; Andrew et al.[2002]). 선박 수중소음은 주로 저주파 대역(< 1 kHz)에서 발생하며 장파장의 저주파 소음은 발생원으로부터 수십 킬로미터를 이동할 수 있어 선박 수중소음은 넓은 지역에 영향을 미칠 수 있다(Urick[1983]). 또한 선박 수중소음의 주파수 대역은 해양 포유류, 어류 및 무척추동물의 가청 주파수 대역과 겹쳐 광범위한 생물 분류군에 영향을 미칠 수 있다(Duarte et al.[2021]; Ferrier-Pagès et al.[2021]). 해양 생물들은 특정 주파수 대역의 음향신호를 활용하여 상호간의 의사소통, 위치파악, 정보수집 등을 하는데 만약 생물학적으로 소리 신호가 더 큰 소음 혹은 유사한 주파수 대역의 소음으로 인해 가려지면 해양 생물들에게 “마스킹 효과(masking effect)”가 발생하게 된다. 그 결과로 짝짓기, 먹이 활동, 포식자 회피가 저해되고 유영 속도 증감, 무리 행동 감소 등의 행동 반응과 호흡 증감, 효소 활성 및 호르몬 변화 등의 생리 반응에 부정적인 영향을 받을 수 있다(Slabbekoorn et al.[2010]; Duarte et al.[2021]).
COVID-19 전 선박통행이 활발했던 지역에서는 COVID-19 전후 수증 음압레벨 비교로 선박운행이 수중음향 환경에 끼치는 영향을 정량화하는 연구가 수행되었다(Breeze et al.[2021]; Thomson and Barclay.[2020]; Dahl et al.[2021]; Gabriele et al.[2021]; Dunn et al.[2021]; Basan et al.[2021]; Bertucci et al.[2021]; Pine et al.[2021]). 하지만 대부분의 연구는 COVID-19 전후의 수중 음향환경 변화에만 치중되어 있으며 이러한 변화가 생물에 미치는 영향에 관한 연구는 미흡한 실정이다. 따라서 본 연구에서는 선박 수중소음에 의한 국내외 어류 및 무척추동물의 영향 범위와 COVID-19 팬데믹 이후 수중 음향환경 변화를 리뷰한 후 선박 운행 제한이 생물에 끼친 영향을 고찰하고 향후 관리 방안에 대해 제언하고자 한다.
2. 재료 및 방법
본 연구에서는 선박 수중소음이 어류 및 무척추동물에 미치는 영향 관련 선행연구를 수집하여 분석하였다. 먼저 학술 데이터 베이스인 “Google Scholar” 및 “Scopus”에서 “어류(fish)”, “무척추동물(invertebrate)”, “선박 소음(vessel noise)”, “배 소음(ship noise)”, “보트 소음(boat noise)”, “인위적 소음(anthropogenic noise)”, “스트레스(stress)”, “영향(impact)”, “행동(behavior)”, “생리(physiological), “반응(responses)”의 키워드로 검색하였고 총20개의 연구논문을 수집하였다. 이후 어류(경골어류, Actinopterygii; 연골어류, Chondrichthyes) 및 무척추동물(연갑류, Malacostraca; 이매패류, Bivalvia; 거미불가사리류; Ophiuroidea)에 대한 선박 수중소음(보트, boat; 배, ship; 선박, vessel)의 노출 영향을 생리(physiology) 및 행동(behavior) 측정항목으로 분류하여 정리하였다(Fig. 1A–C). 선박, 배, 보트에서 발생하는 수중소음은 “선박 수중소음”이라고 통칭한다. 선박 수중소음과 같은 연속음(continuous noise)은 주로 실효(root mean square) 음압레벨(sound pressure level, SPL; 단위: dB re 1 μPa)로 측정되며 본 논문에서는 생물이 측정항목에 유의한 변화를 보이는 음압레벨을 리뷰하여 생물영향을 정량적으로 비교하고자 하였다.
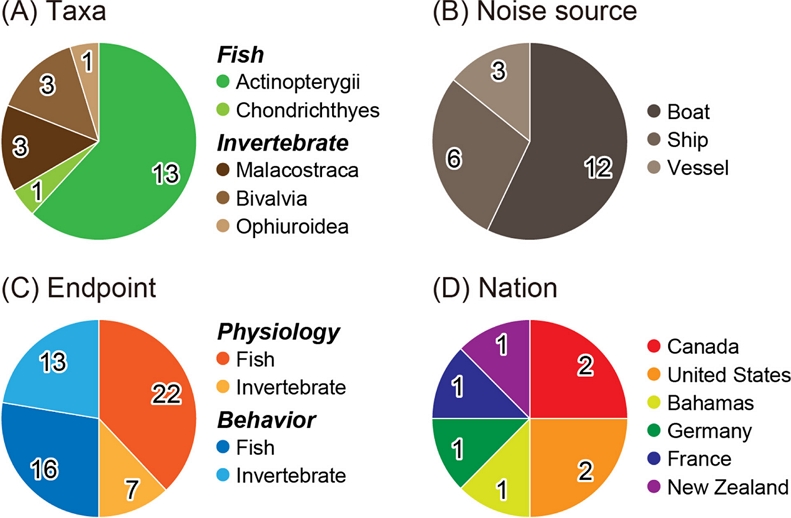
The number of (A) taxa (species level), (B) noise source, and (C) endpoint in the studies assessing the effect of underwater noise on marine fish and invertebrate. The number of (D) nation where changes in underwater soundscape between pre- and during-COVID-19 were investigated.
앞선 방법과 동일하게 COVID-19 전후의 수중 음향환경 변화 관련 선행연구를 수집하여 분석하였다. “COVID-19”, “봉쇄령(lockdown)”, “선박 소음(vessel noise)”, “배 소음(ship noise)”, “보트 소음(boat noise)”, “영향(impact)”, “수중 음향환경(underwater soundscape)”의 키워드를 검색하였고 총 8개의 연구논문을 수집하였다. 6개국(캐나다, 미국, 바하마, 독일, 프랑스)에 대해 COVID-19 전(pre-COVID-19)과 후(during-COVID-19)로 분석 기간을 구분하였고 해당 기간 동안 나타난 음압레벨 차이를 분석하였다(Fig. 1D).
3. 결과 및 고찰
3.1 선박 수중소음이 어류에 미치는 영향
어류의 생물영향을 나타내는 선박 수중소음 크기는 101-148 dB re 1 μPa의 범위로 나타났다(Table 1 그리고 Fig. 2). 어류의 생리적 영향은 호르몬(hormone), 효소활성(enzymatic activity), 호흡(respiration), 청각(hearing), 발달(development) 항목을 측정하여 분석되었다. 이를 확인하기 위해 도미과(Sparus aurata), 두꺼비고기과(Halobatrachus didactylus), 뱀장어과(Anguilla anguilla), 망둥어과(Gobiusculus flavescens), 민어과(Sciaena umbra, Argyrosomus regius)가 사용되었다. 스트레스 상황에 노출되면 어류는 일련의 스트레스 반응을 보이는데 이를 1–3차로 나눌 수 있다. 어류가 위협을 감지하면 1차 스트레스 반응으로 카테콜아민, 코르티솔 등의 호르몬 수치가 급격하게 상승한다. 2차 반응으로 호르몬에 의한 신진대사, 호흡, 면역 기능 변화가 포함되고 3차 반응은 성장, 행동, 면역, 생존 등 개체 전반의 변화이다(Barton, 2002).
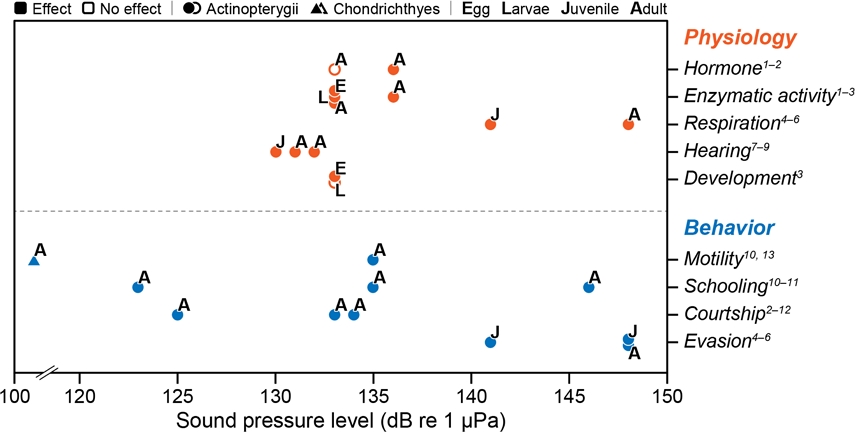
Physiological and behavioral effects of vessel noise on marine fish. Symbol indicates the taxa (circle = Actinopterygii, triangle = Chondrichthyes) and significance of effect (filled = significant, blank = not significant). Letters indicates the developmental stage of fish (E = egg, L = larvae, J = juvenile, A = adult).
1차 스트레스 반응으로 S. aurata은 123–136 dB re 1 μPa의 보트 소음에서 부신피질자극 호르몬과 코르티솔이 유의하게 증가하였으며 H. didactylus는 104–133 dB re 1 μPa의 보트 소음에서 코르티솔이 유의하게 증가하였다(Celi et al.[2016]; Amorim et al.[2022]). 2차 스트레스 반응으로 어류의 전자전달계(electron transport system) 활성, 호흡(환수율, ventilation rate; 아가미 개폐율, opercular beat rate; 산소소비율, oxygen consumption rate) 그리고 청각(청력 민감도, auditory sensitivity, hearing sensitivity; 가청 역치, hearing thereshold)이 측정되었다. 먼저 전자전달계 활성에서 H. didactylus는 어란의 경우 유의하게 감소한 반면, 자어의 경우 유의한 차이가 없어 발달 단계에 따라 결과에 차이를 보였다(Faria et al.[2022]). 어란 및 자치어의 경우 성어에 비해 외부 자극에 대한 민감도가 더높아 수중소음에 의한 생리학적 영향을 알아보는데 비교적 적합할 것으로 사료된다. 호흡 측정결과, A. anguilla는 139–141 dB re 1 μPa 및 148 dB re 1 μPa의 배 소음에서 증가하였다(Bruintjes et al.[2016]; Purser et al.[2016]; Simpson et al.[2015]). 청각의 경우, 130–132 dB re 1 μPa의 보트 소음 노출 시 C. chromis, S. umbra, G. flavescens, H. didactylus, A. regius는 감소하였다(Codarin et al.[2009]; Vasconcelos et al.[2007]; Vieira et al.[2021]).
3차 스트레스 반응으로 어류의 행동적 영향은 유영행동(motility), 무리행동(schooling), 번식행동(courtship), 회피반응(evasion) 항목이 측정되었다. 실험생물로는 뱀장어과(A. anguilla), 참다랑어(Thunnus thynnus), 청어(Clupea pallasii), 연어(Oncorhynchus keta), 곱사연어(Oncorhynchus keta), 두꺼비고기과(H. didactylus), 망둥어과(Gobiusculus flavescens; Pomatoschistus pictus), 두툽상어과(Syliorhinus canicular)가 사용되었다. 먼저 유영행동으로 A. anguilla와 참다랑어는 각각 148 dB re 1 μPa 및 135 dB re 1 μPa 미만의 선박 수중소음 노출 시 유영방향에 유의한 변화가 나타났다(Simpson et al.[2015]; Sarà et al.[2007]). 또한 S. canicular에서 101–135 dB re 1 μPa의 보트 소음 노출 시 유영시간이 유의하게 증가하였다(de Vincenzi et al.[2021]). 참다랑어는 135 dB re 1 μPa 미만, 연어와 곱사연어는 146 dB re 1 μPa의 보트 소음에서 각각 무리활동이 감소하였다(Sarà et al.[2007]; van der Knaap et al.[2022). 행동 측정항목 중 번식행동 범주에는 청각/시각적 구애행동, 번식성공률 등이 포함된다. 번식기에 수컷이 소리를 발생시켜 구애행동을 하는 H. didactylus는 139–141 dB re 1 μPa에서 소리 발생률과 번식성공률이 감소하였다(Amorim et al.[2022]; de Jong et al.[2018]). 선박 수중소음은 어류의 회피반응에도 영향을 끼쳤는데 A. anguilla 에서 139–141 dB re 1 μPa 보트소음 및 148 dB re 1 μPa의 배 소음 노출 시 반포식자반응(anti-predator response)으로 놀람 반응(startle reaction)이 지연되거나 감소하였다(Bruintjes et al.[2016]; Purser et al.[2016]). 놀람반응은 잠재적 위험을 인지하고 방어 연쇄작용을 시작하는 첫 단계이므로 소음 노출 후 놀람 반응이 원활하지 않으면 적절한 방어를 하지 못해 포식자로부터 취약해 질 수 있다(Cacioppo et al.[2007]).
선박 수중소음은 어류에서 생리 및 행동 반응을 아울러 1–3차 스트레스 반응을 모두 유발했으며 선박 수중소음으로 인한 번식행동과 반포식자반응 감소는 향후 개체군 감소의 원인으로 작용될 수 있다. 하지만 한 개체군의 반포식자반응으로 피식률이 높아진다고 볼 수 없기 때문에 해당 포식자의 소음 영향이 함께 고려되어야 한다. 추후 포식자와 피식자 복합 노출 실험으로 포식자-피식자 상호작용을 고려한다면, 소음에 의한 개체군 감소를 예측할 수 있을 것이다. 또한 어류의 생리, 행동 반응을 일련의 메커니즘으로 설명하기 위해서는 생리, 행동 반응을 종합적으로 해석하고 각 측정항목의 상호관계가 상세하게 분석되어야 할 것이다.
3.2 선박 소음이 무척추동물에 미치는 영향
선박 수중소음에 의한 무척추동물 생물영향은 118–155 dB re 1 μPa의 범위로 나타났다(Table 2 그리고 Fig 3). 무척추동물의 생리적 영향은 해당과정 활성(glycolytic activity), 호흡, 발달 항목을 측정하여 분석하였다. 이를 확인하기 위해 가시발새우과(Nephrops norvegicus; Homarus gammarus), 꽃겟과(Carcinus maenas), 바지락(Ruditapes philippinarum), 홍합과(Perna canaliculus), 진주담치(Mytilus edulis), 양편거미불가사리과(Amphiura filiformis)가 사용되었다. 해당과정 활성도는 연갑류, 이매패류, 거미불가사리류에서 각 1종씩 측정되었는데 135–140 dB re 1 μPa의 선박 수중소음 노출 시 모두 유의한 차이를 나타내지 않았다(Solan et al.[2016]). 호흡의 경우, C. maenas는 148–155 dB re 1 μPa의 소음 노출 시 유의하게 증가했다(Wale et al.[2013a]). 선박 수중소음에 의한 유생의 발달영향에서 P. canaliculus는 126 dB re 1 μPa 선박 소음 노출 시 부착 소요 시간 유의하게 감소하였고, 진주담치의 경우, 127 dB re 1 μPa 선박 소음 노출 시 유생 크기가 감소하고 부착률이 유의하게 증가하였다(Wilkens et al.[2012]; Jolivet et al.[2016]). 무척추동물 또한 어류와 마찬가지로 유생이 성체에 비해 외부 자극에 대한 민감도가 더 높아 낮은 음압레벨의 선박 수중소음에도 유의한 차이를 보이는 것으로 사료된다(Fig. 3).
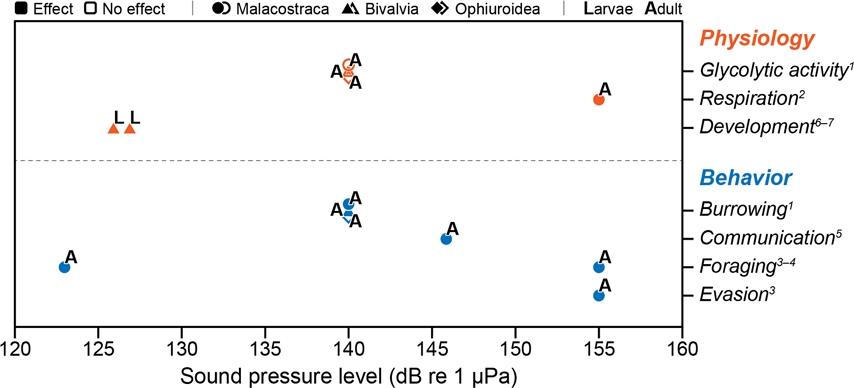
Physiological and behavioral effects of vessel noise on marine invertebrate. Symbol indicates the taxa (circle = Malacostraca, triangle = Bivalvia, diamond = Ophiuroidea) and significance of effect (filled = significant, blank = not significant). Letters indicates the developmental stage of fish (L = larvae, A = adult).
다음으로 무척추동물의 행동 측정항목은 퇴적물을 깊이 파고 들어가는 잠입행동(burrowing), 의사소통(communication), 먹이활동(foragiong), 회피반응(evasion)으로 분류했다. 잠입행동의 경우, 바지락은 135–140 dB re 1 μPa의 소음 노출 시 유의하게 잠입행동이 감소하였다(Solan et al.[2016]). H. gammarus는 갑각진동을 통해 소리(buzzing sound)를 내는데 118–146 dB re 1 μPa의 배 소음에 노출했을 때 갑각진동 횟수가 유의하게 증가하였으나 소리 크기는 감소하는 것으로 나타났다(Jézéquel et al.[2021]). 번식기에 수컷은 갑각진동을 통해 소리를 발생시켜 암컷을 두고 다른 수컷과 우위를 겨루기 때문에 선박 수중소음은 갑각류의 종내 의사소통을 방해하여 번식활동에 부정적인 영향을 미칠 수 있음을 시사한다(Jézéquel et al.[2021]). 먹이반응의 경우, C. maenas는 배 소음 123 dB re 1 μPa, 보트 소음 148–155 dB re 1 μPa에 노출 시 각각 먹이 활동과 먹이 도달 시간에서 유의한 차이를 보였다(Wale et al.[2013b]; Hubert et al.[2021]). 위 결과로 같은 종 내에서 소음원에 따라 영향 범위가 다르게 나타남을 유추할 수 있다.
무척추동물은 분류군 별로 특화된 행동을 보이는 종들이 다수 존재하므로 종별로 행동 측정항목을 세분화하여 선박 수중소음 노출에 대한 생물영향 평가 연구 진행할 필요가 있다. 특히 무척추동물은 어류와 달리 이동반경이 좁고 정착성 종이 많기 때문에 선박 통행이 잦은 항만 및 연안 주변지역에 서식하는 경우 지속적으로 스트레스가 축적되어 어류보다 더 큰 영향을 받을 수 있다. 무척추동물은 대부분 소리가 아닌 진동과 입자운동을 감지하고 반응을 보인다. 갑각류는, 감각모(sensory hair), 평형포, 현음기관(chordotonal organ)에서 이매패류는 평형포, 복부 감각 기관(abdominal sensoorgan)에서 입자운동을 감지한다(Rober and Elliott[2017]). 무척추동물은 어류에 비해 수중소음에 대한 연구가 부족하기 때문에 무척추동물의 생물영향 평가 연구가 더욱 필요할 것으로 사료되며 입자운동 측정에도 초점을 두고 함께 연구해야 할 것이다.
3.3 COVID-19 전후 수중 음향환경 변화
COVID-19 전후 해양환경의 수중소음을 측정한 결과 저주파 대역의 음압레벨이 1.2 dB에서 8.0 dB까지 감소하였으며 평균 3.5 dB 감소된 것으로 보고되었다(Table 3). 감소한 음압레벨 수치는 지역의 환경 요인, 선박과의 근접거리, COVID-19 영향이 발생하기 전 선박의 유형과 수, 봉쇄 강도 및 기간 등에 따라 상이했다. 특히 같은 지역, 동일한 시기에 측정하더라도 조사 정점이 항구 근처에 위치하거나 주요 항로에 있을 경우, 그렇지 않을 때보다 봉쇄 이후 수중소음이 더 많이 감소했다(Dunn et al.[2021]; Pine et al.[2021]; Thomson and Barclay[2020]). 선박 관광이 주를 이루는 관광지의 경우 성수기 때 선박 주운행 시간의 수중소음 최대값을 비교한 결과 COVID-19 전후 차이는 비수기의 경우보다 6.3–7.7 dB이 컸다(Gabriele et al.[2021]; Basan et al.[2021]). 선박 운행이 많은 지역이라 하더라도 수중 청음기가 깊은 수심에 설치된 경우에는 소음원인 선박과의 거리가 멀기 때문에 선박 수중소음 크기가 상대적으로 낮게 측정될 가능성이 있다(Thomson and Barclay[2020]; Dahl et al.[2021]).
COVID-19 전후 수중 음향환경 측정 위치는 4–2189 m 범위로, 대부분 50 m 전후 수심에서 측정하였다(Table 3). 장기간 모니터링을 하는 경우 비교적 깊은 수심에서 하이드로폰을 계류하여 선박 수중소음을 측정하는 경우가 많았다. 생물영향 실험을 위하여 선박 수중소음을 녹음하거나 측정할 경우 평균 5 m 내외에서 수행되므로 COVID-19 전후로 상대적으로 깊은 수심에서 측정한 값과 비교 및 대입하여 생각할 경우 COVID-19 이후 감소된 선박 수중소음이 축소 해석될 수 있다. 저주파 대역의 주변소음은 수심에 큰 영향을 받으며 일반적으로 수심이 증가함에 따라 주변소음이 감소하는 실측결과를 보인다(Marshall[2005]). COVID-19 전후의 선박운행량을 비교해보면 사람이 탑승하는 여객선, 유람선 등의 비율이 가장 크게 감소했고 봉쇄 조치가 끝난 이후에도 가장 운행량 복구가 더뎠다(Breeze et al.[2021]; March et al.[2021]). 서식지가 잘 보존되어 있고 생물 종 다양성이 높은 해양 국립공원에서는 관광 목적의 선박 활동이 많은데 거리두기로 인한 유람선 운행 중단 및 감소는 해당 지역 서식생물들의 건강에 긍정적 영향을 끼쳤을 가능성이 높다(Ryan et al.[2021]). 실제로 COVID-19 발생 이후 선박이 감소함에 따라 어류의 소통 범위 및 빈도가 확장되는 결과도 관찰되었다(Pine et al.[2021]; Bertucci et al.[2021]) 따라서 COVID-19로 인한 수중소음 감소는 생물들에게 긍정적으로 작용했을 가능성이 높다고 판단된다.
COVID-19 전후로 국내 선박 교통량을 비교하기 위해 해운항만물류정보시스템(Port Management Information System, PORT-MIS)에서 3년간(2019–2021년)의 국내 월별 선박 입출항 수 데이터를 이용하였다. 2020년 4월, COVID-19의 영향이 본격화된 이후와 COVID-19 발생 전(2019년)과 비교했을 때 총 선박 입출항 수가 감소하는 경향을 보였다(Fig. 4). 하지만 2021년 총 선박 입출항 수가 증가하며 2020년과 비교해 회복세를 보이는 것으로 보아 세계 경제가 완전히 회복되면 총 선박 입출항 수도 COVID-19 발생 전 수준으로 돌아갈 것으로 예상된다. Kim et al.[2020]은 대한민국 동해 연안의 실제 주변소음 측정값과 인근 항만의 선박 통행량, 해양기상정보 공공데이터를 이용하여 각 데이터의 영향성을 분석하였고 선박 통행량이 수중 주변소음 준위 변화 측면에 있어 가장 큰 요인으로 작용함을 확인하였다. 따라서 국내 2019–2021년 선박 입출항 흐름을 보았을 때 국내 선박 수중소음 역시 그에 상응하는 COVID-19의 영향을 받았을 것이라 사료된다.
3.4 향후 관리방안
본 연구 자료를 기반으로 살펴본 어류 및 무척추동물의 선박 수중소음에 대한 최소 영향 범위는 어류 101–148 dB re 1 μPa, 연갑류 118–155 dB re 1 μPa, 이매패류 126–140 dB re 1 μPa, 거미불가사리류 135–140 dB re 1 μPa이다. 선박 수중소음이 생물에 끼치는 영향이 자명한 만큼 선박 수중소음의 영향을 최소화하기 위해서는 국내 실정에 맞는 선박 수중소음 관리규제를 만들 필요가 있다. 이를 위해서는 국내의 대표적인 수산 양식종, 고유종에 대한 선박 수중소음 영향을 분석하는 연구가 필요하다고 판단된다. 현재 대부분의 수중소음 실험은 개체 수준의 단일 종을 대상으로 많이 수행되는데 향후 개체군, 군집 수준으로 연구 범위를 확장하여 수중소음의 생태학적인 영향을 고려해야 할 것이다. 또한 현장 적용을 위해서는 실제 환경에서의 실험이 진행되어야 할 것이다.
국제해사기구(International Maritime Organization, IMO) 산하의 해양환경보호위원회(Marine Environment Protection Committee, MEPC)에서는 산박 수중소음 및 선박 수중소음이 해양생태계에 미치는 악영향을 의제로 체택하여 규제 논의를 진행중이다. 국내에서는 선박 수중소음 측정, 분석방법을 규격화하고 대형 선박에서 발생하는 소음을 저감하고자 저소음 추진기 설계기술을 개발하였다(KIOST[2019]). 또한 한국선급(Korean Register, KR)은 선박의 수중 방사 소음 감소를 장려하기 위하여 “수중 방사 소음 지침”을 2021년 발행하였다(KR[2021]). 한편, 국내외로 선박자동식별장치(Automatic Identification System, AIS)를 활용하여 선박 수중소음을 추정하는 모델링 방안에 대한 연구가 진행되고 있다(Park et al.[2018]; Porter and Henderson[2014]). 미국 해양대기청(National Oceanic and Atmospheric Administration, NOAA)은 전세계 평균 연간 선박 소음지도를 만들었다. 따라서 국내에서도 선박 소음지도 제각과 같은 기술개발이 지속적으로 진행된다면 선박 소음이 집중된 특정 해역의 경우 생물영향 평가가 수행되고 해당 해역에 서식하는 어류에 선박 소음이 미친 영향에 대해 논의가 이루어질 것이다. 또한 생물 다양성이 풍부한 해양보호구역이나 해상 어장 같은 경우 공간관리 체제를 이용하여 선박 및 선박 수중소음을 관리하고 해당 해역에 서식하는 생물 보호가 필요할 것으로 사료된다.
4. 결 론
본 연구에서는 국내외 선행연구 리뷰를 통해 선박 소음에 대한 어류 및 무척추동물의 영향 범위를 산정하고 COVID-19로 인한 선박 수중소음 감소 사례를 정리하였다. 어류 13종, 무척추동물 7종에 대하여 생리 및 행동 반응을 나누어 분석하였다. 그 결과 118–155 dB re 1 μPa의 선박 수중소음 노출 시 유의하게 반응하였다. COVID-19 이후 봉쇄 조치로 6개국에서 수중 음향환경의 음압레벨이 1.2–8.0 dB re 1 μPa 감소하였다. 장기간 수중 음향환경을 모니터링할 경우 수심 깊은 곳에 수중청음기를 계류하여 측정하기 때문에 수면 가까이에서 측정된 값과 비교하면 과소평가될 가능성이 있다.
위 결과를 통해 COVID-19 이후 선박 수중소음 감소는 해양 생물들에게 긍정적으로 작용했을 가능성이 높다고 판단된다. COVID-19 이후, 또한 국내에서도 총 선박 입출항 수가 감소하였기에 COVID-19 전 선박 운행이 집중된 해역의 경우 해당 지역 및 주변 생물의 건강에 긍정적으로 작용했을 것이라고 예상할 수 있다. 하지만 수중 소음 감소는 일시적일 뿐 세계 경제 및 선박 운행률이 회복되면 선박 수중소음 발생이 이전과 같이 다시 증가할 것으로 사료된다. 따라서 선박 수중소음으로부터 생물 영향을 최소화하기 위한 국내 실정에 맞는 관리방안 마련이 시급하다고 판단되며 이를 뒷받침할 수 있는 과학적인 근거 마련이 필요하다.
Acknowledgments
본 논문은 2023년도 정부(해양수산부)의 재원으로 해양수산과학기술진흥원-과학기술기반 해양환경영향평가 기술개발 사업 지원을 받아 수행된 연구임(KIMST-20210427).
References
-
Amorim, M.C.P., Vieira, M., Meireles, G., Novais, S.C., Lemos, M.F., Modesto, T., Alves, D., Zuazu, A., Lopes, A.F., Matos, A.B. and Fonseca, P.J., 2022, Boat noise impacts Lusitanian toadfish breeding males and reproductive outcome, Sci. Total Environ., 830, 154735.
[https://doi.org/10.1016/j.scitotenv.2022.154735]
-
Andrew, R.K., Howe, B.M., Mercer, J.A. and Dzieciuch, M.A., 2002, Ocean ambient sound: comparing the 1960s with the 1990s for a receiver off the California coast, Acoust. Res. Lett. Online, 3(2), 65-70.
[https://doi.org/10.1121/1.1461915]
-
Barton, B.A., 2002. Stress in fishes: A diversity of responses with particular reference to changes in circulating corticosteroids. Integr. Comp. Biol., 42(3), 517-525.
[https://doi.org/10.1093/icb/42.3.517]
-
Basan, F., Fischer, J.G. and Kühnel, D., 2021, Soundscapes in the German Baltic Sea before and during the COVID-19 Pandemic, Front. Mar. Sci., 8, 689860.
[https://doi.org/10.3389/fmars.2021.689860]
-
Bertucci, F., Lecchini, D., Greeven, C., Brooker, R.M., Minier, L., Cordonnier, S., Ren´e-Trouillefou, M. and Parmentier, E., 2021, Changes to an urban marina soundscape associated with COVID-19 lockdown in Guadeloupe, Environ. Pollut., 289, 117898.
[https://doi.org/10.1016/j.envpol.2021.117898]
-
Braga, F., Scarpa, G.M., Brando, V.E., Manfè, G. and Zaggia, L., 2020, COVID-19 lockdown measures reveal human impact on water transparency in the Venice Lagoon. Sci. Total Environ., 736, 139612.
[https://doi.org/10.1016/j.scitotenv.2020.139612]
-
Breeze, H., Li, S., Marotte, E.C., Theriault, J.A., Wingfield, J. and Xu, J., 2021, Changes in Underwater Noise and Vessel Traffic in the Approaches to Halifax Harbor, Nova Scotia, Canada, Front. Mar. Sci., 8, 674788.
[https://doi.org/10.3389/fmars.2021.674788]
-
Bruintjes, R., Purser, J., Everley, K.A., Mangan, S., Simpson, S.D. and Radford, A.N., 2016, Rapid recovery following short-term acoustic disturbance in two fish species, R. Soc. Open Sci., 3(1), 150686.
[https://doi.org/10.1098/rsos.150686]
- Cacioppo, J.T., Tassinary, L.G. and Berntson, G., 2007, Handbook of psychophysiology, third ed., Cambridge university press, New York.
-
Callejas, I.A., Lee, C.M., Mishra, D.R., Felgate, S.L., Evans, C., Carrias, A., Rosado, A., Griffin, R., Cherrington, E.A., Ayad, M., Rudresh, M., Page, B.P. and Jay, J.A., 2021, Effect of COVID-19 Anthropause on water clarity in the Belize coastal lagoon, Front. Mar. Sci., 8, 648522.
[https://doi.org/10.3389/fmars.2021.648522]
-
Celi, M., Filiciotto, F., Maricchiolo, G., Genovese, L., Quinci, E.M., Maccarrone, V., Mazzola, C., Vazzazana, M. and Buscaino, G., 2016, Vessel noise pollution as a human threat to fish: assessment of the stress response in gilthead sea bream (Sparus aurata, Linnaeus 1758), Fish Physiol. Biochem., 42(2), 631-641.
[https://doi.org/10.1007/s10695-015-0165-3]
-
Codarin, A., Wysocki, L.E., Ladich, F. and Picciulin, M., 2009, Effects of ambient and boat noise on hearing and communication in three fish species living in a marine protected area (Miramare, Italy), Mar. Pollut. Bull., 58(12), 1880-1887.
[https://doi.org/10.1016/j.marpolbul.2009.07.011]
-
Dahl, P.H., Dall'Osto, D.R. and Harrington, M.J., 2021, Trends in low-frequency underwater noise off the Oregon coast and impacts of COVID-19 pandemic, J. Acoust. Soc. Am., 149(6), 4073-4077.
[https://doi.org/10.1121/10.0005192]
-
de Jong, K., Amorim, M.C.P., Fonseca, P.J., Fox, C.J. and Heubel, K. U., 2018, Noise can affect acoustic communication and subsequent spawning success in fish, Environ. Pollut., 237, 814-823.
[https://doi.org/10.1016/j.envpol.2017.11.003]
-
de Vincenzi, G., Micarelli, P., Viola, S., Buffa, G., Sciacca, V., Maccarrone, V., Corrias, V., Reinero, F.R. Giacoma, C. and Filiciotto, F., 2021, Biological sound vs. Anthropogenic noise: Assessment of behavioural changes in Scyliorhinus canicula exposed to boats noise, Animals, 11(1), 174.
[https://doi.org/10.3390/ani11010174]
-
Duarte, C.M., Chapuis, L., Collin, S.P., Costa, D.P., Devassy, R.P., Eguiluz, V.M., Erbe, C., Gordon, T.A.C., Halpern, B.S., Harding, H.R., Havlik, M.N., Meekan, M., Merchant, N.D., Miksis-Olds, J.L., Parsons, M., Predragovic, M., Radford, A.N., Radford, C.A., Simpson, S.D., Slabbekoorn, H., Staaterman, E., Van Opzeeland, I.C., Winderen, J., Zhang, X. and Juanes, F., 2021, The soundscape of the Anthropocene ocean, Science, 371(6529), eaba4658.
[https://doi.org/10.1126/science.aba4658]
-
Dunn, C., Theriault, J., Hickmott, L. and Claridge, D., 2021, Slower ship speed in the Bahamas due to COVID-19 produces a dramatic reduction in ocean sound levels, Front. Mar. Sci., 8, 673565.
[https://doi.org/10.3389/fmars.2021.673565]
-
Faria, A., Fonseca, P.J., Vieira, M., Alves, L.M.F., Lemos, M.F.L., Novais, S.C., Matos, A.B., Vieira, D. and Amorim, M.C.P., 2022, Boat noise impacts early life stages in the Lusitanian toadfish: A field experiment, Sci. Total Environ., 811, 151367.
[https://doi.org/10.1016/j.scitotenv.2021.151367]
-
Ferrier-Pagès, C., Leal, M.C., Calado, R., Schmid, D.W., Bertucci, F., Lecchini, D. and Allemand, D., 2021, Noise pollution on coral reefs? —A yet underestimated threat to coral reef communities, Mar. Pollut. Bull., 165, 112129.
[https://doi.org/10.1016/j.marpolbul.2021.112129]
-
Frisk, G.V., 2012, Noiseonomics: The relationship between ambient noise levels in the sea and global economic trends, Sci. Rep., 2(1), 1-4.
[https://doi.org/10.1038/srep00437]
-
Gabriele, C.M., Ponirakis, D.W. and Klinck, H., 2021, Underwater sound levels in Glacier Bay during reduced vessel traffic due to the COVID-19 pandemic, Front. Mar. Sci., 8, 674787.
[https://doi.org/10.3389/fmars.2021.674787]
-
Hubert, J., van Bemmelen, J.J. and Slabbekoorn, H., 2021, No negative effects of boat sound playbacks on olfactory-mediated food finding behaviour of shore crabs in a T-maze, Environ. Pollut., 270, 116184.
[https://doi.org/10.1016/j.envpol.2020.116184]
-
Jézéquel, Y., Bonnel, J. and Chauvaud, L., 2021, Potential for acoustic masking due to shipping noise in the European lobster (Homarus gammarus), Mar. Pollut. Bull., 173, 112934.
[https://doi.org/10.1016/j.marpolbul.2021.112934]
-
Jiang, Q., Xu, Z., Ye, G., Pahlow, M., Hu, M. and Qu, S., 2022, A systematic scoping review of environmental and socio-economic effects of COVID-19 on the global ocean-human system, Sci. Total Environ., 849, 157925.
[https://doi.org/10.1016/j.scitotenv.2022.157925]
-
Jolivet, A., Tremblay, R., Olivier, F., Gervaise, C., Sonier, R., Genard, B. and Chauvaud, L., 2016, Validation of trophic and anthropic underwater noise as settlement trigger in blue mussels, Sci. Rep., 6(1), 1-8.
[https://doi.org/10.1038/srep33829]
- Kim, Y.G., Kook, Y.M., Kim, D.G., Kim, K., Youn, S.K., Choi, C.H. and Kim, H.K., 2020, Analysis of the influence of ship traffic and marine weather information on underwater ambient noise using public data, J. Acoust. Soc. Kr., 39(6), 606-614.
- KIOST (Korea Institute of Ocean Science & Technology), 2019, Planning Study for Construction of Public Certification Measurement Center of Shipping Noise to Response to IMO Regulation.
- KR (Korean Register), 2021, Guidances for Underwater Radiated Noise.
-
March, D., Metcalfe, K., Tintoré, J. and Godley, B.J., 2021, Tracking the global reduction of marine traffic during the COVID-19 pandemic, Nat. Commun., 12(1), 1-12.
[https://doi.org/10.1038/s41467-021-22423-6]
- Park, J.S., Kang, D., Kim, H., Kim, M. and Cho, S, 2018, A study on the estimation of underwater shipping noise using automatic identification system data, J. Acoust. Soc. Kr., 37(3), 129-138.
-
Pine, M.K., Wilson, L., Jeffs, A.G., McWhinnie, L., Juanes, F., Scuderi, A. and Radford, C.A., 2021, A Gulf in lockdown: how an enforced ban on recreational vessels increased dolphin and fish communication ranges, Global Change Biol., 27(19), 4839-4848.
[https://doi.org/10.1111/gcb.15798]
- Porter, M.B. and Henderson, L.J., 2014, Modeling ocean noise in global scale, in proc. of INTER-NOISE and NOISE-CON Congress and Conference Proceedings, Institute of Noise Control Engineering, 249(1), 6112-6118.
-
Purser, J., Bruintjes, R., Simpson, S.D. and Radford, A.N., 2016, Condition-dependent physiological and behavioural responses to anthropogenic noise, Physiol. Behav., 155, 157-161.
[https://doi.org/10.1016/j.physbeh.2015.12.010]
-
Roberts, L. and Elliott, M., 2017, Good or bad vibrations? Impacts of anthropogenic vibration on the marine epibenthos, Sci. Total Environ., 595, 255-268.
[https://doi.org/10.1016/j.scitotenv.2017.03.117]
-
Ryan, J.P., Joseph, J.E., Margolina, T., Hatch, L.T., Azzara, A., Reyes, A., Southall, B.L., DeVogelaere, A., Reeves, L.E.P., Zhang, Y., Cline, D.E., Jones, B., McGill, P., Baumann-Pickering, S. and Stimpert, A.K., 2021, Reduction of low-frequency vessel noise in Monterey Bay National Marine Sanctuary during the COVID-19 pandemic, Front. Mar. Sci., 8, 656566.
[https://doi.org/10.3389/fmars.2021.656566]
-
Sarà, G., Dean, J.M., d’Amato, D., Buscaino, G., Oliveri, A., Genovese, S., Ferro, S., Buffa, G. and Mazzola, S., 2007, Effect of boat noise on the behaviour of bluefin tuna Thunnus thynnus in the Mediterranean Sea, Mar. Eco. Prog. Ser., 331, 243-253.
[https://doi.org/10.3354/meps331243]
-
Simpson, S.D., Purser, J. and Radford, A.N., 2015, Anthropogenic noise compromises antipredator behaviour in European eels, Global Change Biol., 21(2), 586-593.
[https://doi.org/10.1111/gcb.12685]
-
Slabbekoorn, H., Bouton, N., van Opzeeland, L., Coers, A., Cate, C.T. and Popper, A.N., 2010, A noisy spring: the impact of globally rising underwater sound levels on fish, Trends Ecol. Evol., 25(7), 419-427.
[https://doi.org/10.1016/j.tree.2010.04.005]
-
Solan, M., Hauton, C., Godbold, J.A., Wood, C.L., Leighton, T.G. and White, P., 2016, Anthropogenic sources of underwater sound can modify how sediment-dwelling invertebrates mediate ecosystem properties, Sci. Rep., 6(1), 1-9.
[https://doi.org/10.1038/srep20540]
-
Thomson, D.J. and Barclay, D.R., 2020, Real-time observations of the impact of COVID-19 on underwater noise, J. Acoust. Soc. Am., 147(5), 3390-3396.
[https://doi.org/10.1121/10.0001271]
- Urick, R.J., 1983, Principles of underwater sound, third ed., McGraw-Hill, New York.
-
Vasconcelos, R.O., Amorim, M.C.P. and Ladich, F., 2007, Effects of ship noise on the detectability of communication signals in the Lusitanian toadfish, J. Exp. Biol., 210(12), 2104-2112.
[https://doi.org/10.1242/jeb.004317]
-
Vieira, M., Beauchaud, M., Amorim, M.C.P. and Fonseca, P.J., 2021, Boat noise affects meagre (Argyrosomus regius) hearing and vocal behavior, Mar. Pollut. Bull., 172, 112824.
[https://doi.org/10.1016/j.marpolbul.2021.112824]
-
Wale, M.A., Simpson, S.D. and Radford, A.N. 2013, Size-dependent physiological responses of shore crabs to single and repeated playback of ship noise, Biol. Lett., 9(2), 20121194.
[https://doi.org/10.1098/rsbl.2012.1194]
-
Wale, M.A., Simpson, S.D. and Radford, A.N., 2013, Noise negatively affects foraging and antipredator behaviour in shore crabs, Anim. Behav., 86(1), 111-118.
[https://doi.org/10.1016/j.anbehav.2013.05.001]
-
Wilkens, S.L., Stanley, J.A. and Jeffs, A.G., 2012, Induction of settlement in mussel (Perna canaliculus) larvae by vessel noise, Biofouling, 28(1), 65-72.
[https://doi.org/10.1080/08927014.2011.651717]